
Drying Techniques and Nutritional Composition of Drone Pupae (Apis mellifera L.) as Edible Food
Abstract
There is an urgent need for novel protein sources as an alternative to meat production. Insects, such as honeybees, hold potential as a safe, nutritious and reliable protein source for the future. In the present study, we established optimal powder preprocessing conditions of drone pupae (Apis mellifera L.) for use as a novel food. The content of moisture, crude protein, crude fat, crude ash, carbohydrate and crude fiber in drone pupae (Apis mellifera L.) were analyzed. The crude protein content ranged from 48.5 to 51.8% was found in both freeze-dried and hot-air powdered drone pupae. However, the protein content in the freeze-dried powder was higher than that in the hot-air powder by 3.3%. According to the Korean Food Standard Codex test method, coliforms, Salmonella spp. Staphylcoccus aureus, and Enterohamorrhagice Escherichia coli were not detected in both freeze-dried and hot-air powder. Therefore, we suggest that the high protein content of the powdered drone pupae prepared in this study can serve as a novel food.
Keywords:
Apis mellifera, Drone pupae, Freeze-drying, Harmful microorganisms, Hot-air drying, Nutritional valueINTRODUCTION
The honey bee is an insect that produces useful apiculture products (was, honey, propolis, bee venom, and royal jelly) for humans. In South Korea, the species Apis mellifera L. is most commonly cultured (Ghisalberti, 1979). However, as global warming reduces the production of acacia honey, the main source of income for beekeeping farms, a new income source needs to be developed. The Food and Agricultural Organization of the United Nations (FAO) has proposed edible insects as an alternative to solve problems with food supply and distribution caused by various factors, including global warming (van Huis et al., 2013). Edible insects are bred for human consumption, with Lepidoptera, Hymenoptera, Coleoptera, Orthoptera, Isoptera, Coleoptera, Orthoptera, and Isoptera as the representative orders (Yoo et al., 2013). These edible insects are valuable nutritional sources containing not only protein but also lipid, dietary fiber, and vitamin B (Bukkens, 1997). Honeybee is one of such insect with potential to become the source of protein. In 2011, the Korean Ministry for Food, Agriculture, Forestry, and Fisheries (Now the Ministry of Agriculture, Food, and Rural Affairs) announced a 5-year comprehensive plan to foster the insect industry and started supporting relevant research projects. Until now, 7 species of edible insects have been registered in the Korean Food Standard Codex of the Ministry of Food and Drug Safety (Lee et al., 2017). Apart from three previously listed species, Bombyx mori L., Oxya japonica Tunberg, and Beauveria bassiana Vuill., four new species, namely Tenebrio molitor L., Protaetia brevitarsis L., Allomyrina dischotoma L, and Gryllus bimaculatus L, have been added as food materials since 2014 (MFDS, 2016). The honeybee is an insect that produces useful apiculture products (honey, propolis, bee venom, and royal jelly) for humans. In South Korea, Apis mellifera L. is mainly bred (Ghisalberti, 1979). However, as global warming reduces the production of acacia honey, the main source of income for beekeeping farms, a new income source needs to be developed.
A recent study showed that drone pupae contain various nutrients such as carbohydrate, fat, protein, essential amino acids, and inorganic matter (Kim et al., 2018). Unlike worker bees, whose chemical composition has been investigated by Ghosh et al. (2016), drones do not contribute much to the colony other than mating with a queen bee. However, drones are easily bred, and there is an established method of producing drones in beekeeping farms. In this regard, drone pupae are expected to generate economic profits if used as a food material and the Swiss food insect enthusiast Daniel Ambuehl has even published a book on drone bees as human food and provided recipes to prepare drones for human consumption.
However, how safe are drones to eat? The FAO reported that honey bees can be a safe food material providing sufficient protein in all their life forms, namely imago, larva, and pupa. In addition, drones have been a traditional source of protein for centuries (FAO, 1996) and various recipes incorporating drones have been developed (Ambuehl, 2017). However, drone pupae have not been registered as a food material in South Korea. Although the safety, nutritional contents, and functions of drone pupae as food have been actively studied, few remarkable findings have been reported in terms of storage and pretreatment of collected drone pupae. To mitigate people’s hesitation to consume insects in their original forms, edible insects may be pretreated into powders and finally consumed as processed foods as suggested as early as 1975 by Meyer-Rochow (1975). Accordingly, the development of various processing methods for enhancing the use of drone pupae as food will be of considerable help in facilitating the value of drone pupae as a food resource.
This study examined the nutritional contents and hygienic quality of collected drone pupae under preprocessing conditions to ensure the safety of this new food material.
MATERIALS AND METHODS
Sampling
In 2018, drone pupae aged 17~23 days old produced by a beekeeping farm in Cheongyang-gun, South Korea were used as testing materials. The drone pupae were stored in a freezer operated at -20°C immediately after collection.
Thawing conditions
Room temperature condition: the frozen drone pupae were thawed at room temperature (18~24°C), they were washed by distilled water to remove impurities. Next, the drone pupae were dehydrated under natural conditions and used as material.
Cold condition: Frozen drone pupae were thawed in a refrigerator (0~4°C) and then washed with distilled water to remove impurities. Next, the drone pupae were dehydrated under natural conditions and used as material.
Drying conditions
Freeze drying: After the drone pupa samples were frozen for over 24 h at -70°C, a freeze dryer (SFDSM24L, Samwon Freezing Engineering Co.) was used to completely remove all water from the samples. The dried samples were then used for the test.
Hot air drying: Samples were dried in a hot-air dryer (JSR, JSOF-150, Forced Convection Oven) operating at 90°C. The dried samples were then used for the test.
Sterilizing condition
Drone pupae were sterilized in a high-pressure sterilizer (Auto-Steam Stetrilizer, VS-1321-80, Vision Scientific Co., Ltd.).
Nutritional composition analysis
The nutritional composition of drone pupae were analyzed in accordance with the testing methods of food components proposed by the Korean Food Standard Codex of the Ministry of Food and Drug Safety. The moisture content of drone pupae was determined by drying the sample in an oven at 105°C and at atmospheric pressure until a constant weight was obtained.
Protein was measured by the Kjeldahl method, where an accelerator for proteolysis and sulfuric acid were added to sample to split proteins, and then protein content was measured by using an automatic analyzer (FOSS kjeltec 8400, Fisher Scientific, Hampton, NH, USA). Crude fat was analyzed by using the Soxhlet method with petroleum ether as an extractant. Crude fat was analyzed by using petroleum ether as an extractant and applying the Soxhlet method. Carbohydrate content was calculated by subtracting the content of crude protein, crude fat, moisture, and ash from a 100-g sample.
Microbiological analysis
For simple microbial detection, the dry film method was applied on defrosted, freeze-dried, and hot-air dried drone pupae. Petrifilm aerobic count plate (3M), Petrifilm coliform count plate (3M), Petrifilm Escherichia coli count plate (3M) were used to detect general microorganisms, E. coli, and molds. General microorganisms and E. coli were incubated in a medium for 24~48 h at 35°C. Molds were incubated for 72 h at 25°C before detection was conducted.
To ensure microbiological stability according to the preprocessing conditions for drone pupae, food poisoning bacteria and coliforms were analyzed according to the provisions of the Korean Food Standard Codex. Coliforms were analyzed in accordance with the BGLB (brilliant green lactose bile broth, Hardy diagnostics, CA, USA) medium method provided by the Korean Food Standard Codex. First, 25 g of drone pupae were placed in 9 times purified water for homogenization. Next, 1 mL of the homogenate was added to BGLB medium, and after a 48 h incubation at 36°C, the presence of coliforms was checked according to the occurrence of BGLB (Hardy diagnostics, CA, USA) gas. Salmonella spp. was also analyzed in accordance with the Salmonella analysis method of the Korean Food Standard Codex. After 25 g of drone pupae were placed in 9 times peptone water primary incubation was performed for 24 h at 36°C. Next, 0.1 mL of culture solution was added to 10 mL of Rappaport-Vassiliadis medium, and the secondary incubation was conducted for 24 h at 42°C. Next, the secondary culture solution was inoculated to XDL (xylose lysine desoxycholate) agar and then incubated for 24 h at 36°C. The formation of pink colony was checked to detect Salmonella spp. Staphylococcus aureus was analyzed by applying the qualitative test method of the Korean Food Standard Codex. Briefly, 25 g of drone pupae were added to 225 mL of TSB (tryptic soy broth) medium, to which 10% sodium chloride solution had also been added. After a 24 h incubation at 36°C, the medium was inoculated to yoke-added mannitol agar, which was then incubated for another 24 h at 36°C. The presence of S. aureus was checked by identifying yellow opaque colonies surrounded by a ring of white opaque circular colonies. Enterohaemorrhagic Escherichia coli were analyzed in accordance with the corresponding method provided by the Korean Food Standard Codex. Briefly, 25 g of drone pupae was added to 225 mL of mTSB (modified tryptone soy broth) medium. After a 24 h incubation at 36°C, 1 mL of the culture solution was isolated and then washed. Next, 200 μL of sterilized water was added to the solution, which was then boiled for 10 min. After that, 5 μL of supernatant was obtained by centrifugation and then used as the template DNA. PCR analysis was conducted by using VT1 and VT2 primers. Electrophoresis was performed using the final reaction solution and 2% agarose, and the reaction products (VT1: 180 bp, VT2: 255 bp) were then identified by using UV to detect the presence of enterohemorrhagic E. coli.
Statistical treatment
In this study, statistical significance of test results were analyzed by using the R (3.4.1 version, New Zealand) statistical program. The significance of differences of the mean values between the control and experimental groups was verified by means of analysis of variance (ANOVA).
RESULTS AND DISCUSSION
Establishment of thawing conditions
In preparing drone pupae as materials for food processing, thawing conditions before preprocessing need to be established to remove impurities from the drone pupae, which were stored in a freezer immediately after collection. It was revealed that thawing for 1 h at room temperature and for 12 h under cold conditions was sufficient to turn the drone pupae into their original form (Fig. 1). Although thawing duration can vary depending on the quantity of the drone pupae to be defrosted, room temperature was advantageous to defrost a small number of drone pupae in a short time, whereas the cold conditions seemed to be advantageous to defrost a large number of drone pupae. Defrosted drone pupae were then cleaned with running water, with the final cleaning round being manually performed two or three times with distilled water. The drone pupae were then put in a tray, and moisture was removed from the pupae (Fig. 2). Dehydration after moisture removal could be performed quickly by using an electric fan, requiring no more than 1 h even at room temperature (Fig. 3).
Nutritional compositions
Table 1 presents the results of the nutritional compositions of drone pupae following different preprocessing conditions. Moisture content was as low as 2.1% and 3.6% after freeze-drying process and hot-air drying processes, respectively. Crude protein content was 51.8% following freeze-drying and 48.5% after hot-air drying. Because of crude protein accounts of 27.7% (pork), 54.7% (chicken) and 71.2% (veal) with regard to conventional meat contents (USDA database) and 10.4~47.7% of fish content, it was obvious that drone pupae possessed a relatively high protein content (MFDS, 2018). Compared with the protein contents of other edible insects such as, Tenebrio molitor and Allomyrina. Dichotoma, whose respective protein contents have been reported as 53.22% and 54.18% (Ghosh et al., 2017) that of drone bee pupae is very similar, although it does not reach the amounts present in Teleogryllus emma (55.65%) and Gryllus bimaculatus (58.32). It is, however, nearly identical to the protein content of adult worker bees (Ghosh et al., 2016). Drone pupae did not show any significant difference in ash, crude fat, and carbohydrate content between freeze-dried and hot-air dried pupae. Thus, it was suggested that both freeze-drying and hot-air drying processes were applicable for processing drone pupae as an alternative to the existing sources of protein rich foods. However, what ultimately matters is not only the amount of protein, but its composition, i.e., the amino acids present. In this regard worker bee protein (as Ghosh et al., 2016 have shown) satisfies the level of all the essential amino acids as recommended by FAO/WHO/UNU (2007) and the same can perhaps be expected to apply to drone bees as well. What is remarkable and needs to be mentioned is the low carbohydrate content of drone bee pupae, which is at least half of that of worker bee larvae and adults (Ghosh et al., 2016).
Analysis of harmful microorganisms
Table 2 presents the detection results of harmful microorganisms in drone bee pupae after different preprocessing conditions. The presence of microorganisms in drone pupae was checked by using a dry film medium. Next, a qualitative analysis was conducted on the drone pupae samples, which had been subjected to either freeze-drying or hot-air drying, to identify coliforms like, Salmonella spp. and, S. aureus, as well as enterohamorrhagic E. coli. Before freeze-drying and hot-air drying, drone pupae samples exhibited bacteria and molds. However, E. coli was not detected in any samples. After freeze-drying and hot-air drying, those samples that had shown bacteria and molds and were then subjected to hot-air drying, did not show the presence of any microorganism, whereas freeze-drying could not totally remove the bacteria (Fig. 4). Accordingly, a high-pressure sterilization process was added to the freeze-drying process to remove bacteria and molds (Fig. 4). The drone pupae samples that were subjected to the high-pressure sterilization process contained no harmful microorganism. Consequently, the freeze-drying process was revealed to be a safe processing method of drone pupae. High-pressure sterilization needs to be conducted before freeze-drying to ensure that drone pupae are safe as a food item and free from harmful microorganisms.
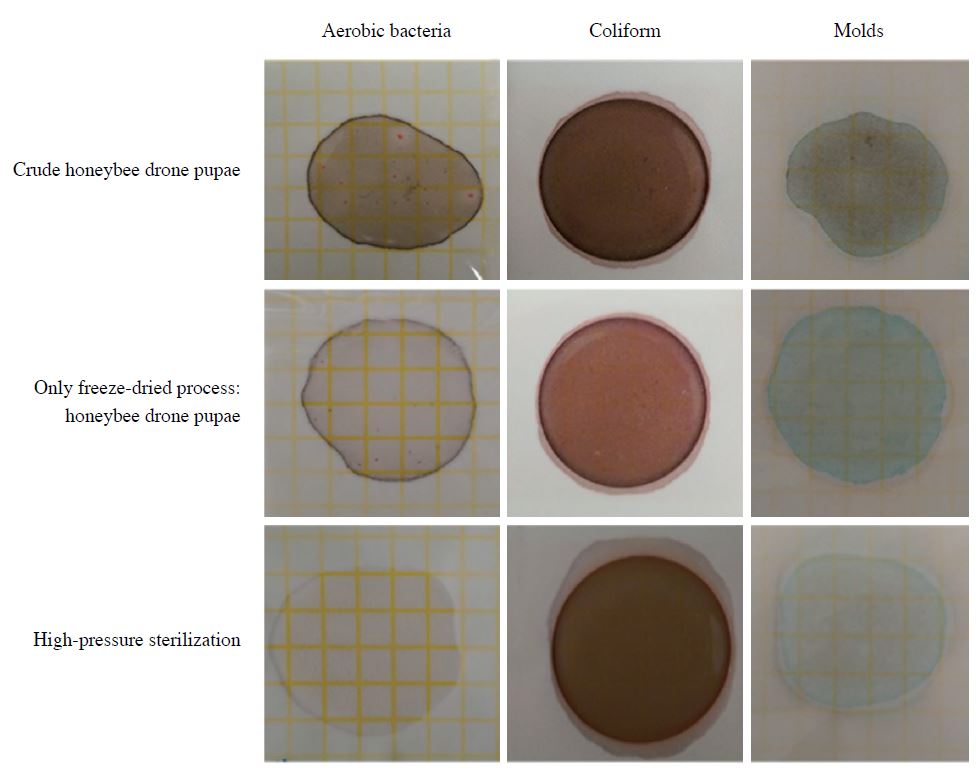
Detecting food poisoning bacteria and molds in honeybee drone pupae by dry rehydratable film following different processing conditions.
We carried out a series of tests to establish preprocessing conditions of honey bee drone pupae as an alternative food item with high nutritional value to be mass-produced by beekeeping farms. For drone pupae that had been stored in a freezer immediately after collection, thawing for 1 h at room temperature and 12 h under cold conditions was sufficient to turn them back into their original form. Freeze-dried drone pupae possessed a slightly higher crude protein content than hot air-dried samples did. However, the levels of other nutrients, such as crude fat and fibre, were similar between the two preprocessing conditions. Regarding the safety of drone pupae, no pathogenic organisms were detected in samples subjected to either hot-air drying of high-pressure sterilization followed by freeze-drying. In conclusion, the drone pupae were found to be rich in its nutritional values, including high content of protein (48.5~51.8%), fatty acids and carbohydrates, indicated that it is an ideal source for food and feeds. Interestingly, the protein content of drone pupae is even better than many edible insects. Utilization of drone pupae would help prevent nutrition deficiencies in developing countries as well as it could become a good source of income for honeybee keepers in Korea. Therefore, further study on the quality of the proteins, which is determined by the amino acid composition and its digestibility, is necessary.
Acknowledgments
This work was supported by the Research Project of Basic Technologies in Agricultural Science (PJ01314202), which was funded by the Rural Development Administration.
References
- Ambuehl, D., (2017), Booza - the kingbee cook book, Selbstverlag, Unterterzen (Switzerland).
-
Bukkens, S. G. F., (1997), The nutritional value of edible insects, Ecol. Food Nutr., 36, p287-319.
[https://doi.org/10.1080/03670244.1997.9991521]
- Food and Agricultural Organization (FAO), (1996), The state of food and agriculture, Roma.
-
Ghisalberti, E. L., (1979), Propolis: a review, Bee World, 60, p59-84.
[https://doi.org/10.1080/0005772x.1979.11097738]
-
Ghosh, S., C. Jung, and V. B. Meyer-Rochow, (2016), Nutritional value and chemical composition of larvae, pupae, and adults of worker honey bee, Apis mellifera ligustica as a sustainable food source, J. Asia Pac. Entomol., 19, p487-495.
[https://doi.org/10.1016/j.aspen.2016.03.008]
-
Ghosh, S., S.-M. Lee, C. Jung, and V. B. Meyer-Rochow, (2017), Nutritional composition of five commercial edible insects in South Korea, J. Asia Pac. Entomol., 20, p686-694.
[https://doi.org/10.1016/j.aspen.2017.04.003]
-
Kim, S. G., S. O. Woo, K. W. Bang, H. R. Jang, and S. M. Han, (2018), Chemical composition of drone pupa of Apis mellifera and its nutritional evaluation, J. Apiculture., 33(1), p17-23.
[https://doi.org/10.17519/apiculture.2018.04.33.1.17]
- Lee, H. S., H. J. Ryu, H. J. Song, and S. O. Lee, (2017), Enzymatic preparation and antioxidant activities of protein hydrolysates from Protaetia brevitarsis Larvae, J. Korean Soc. Food Sci. Nutr., 46(10), p1164-1170.
- Meyer-Rochow, V. B., (1975), Can insects help to ease then problem of world food shortage?, Search, 6, p261-262.
- Ministry of Food and Drug Safety (MFDS), (2016), Revision of Korea Food Code (2016-18).
- Ministry of Food and Drug Safety (MFDS), (2018), Food Code, Korea.
- USDA database on nutrient content of food, d. (Available at:) http://www.ndb.nal.usda.gov, (availed on May, 2013).
- van Huis, A., J. Van Itterbeeck, H. Klunder, E. Mertens, A. Halloran, G. Muir, and P. Vantomme, (2013), Edible insects: future prospects for food and feed security, Food and Agriculture Organization of the United Nations, Rome, Italy.
-
Yoo, J. M., J. S. Hwang, T. W. Goo, and E. Y. Yun, (2013), Comparative analysis of nutritional and harmful components in Korean and Chinese mealworms (Tenebrio molitor), J. Korean Soc. Food Sci. Nutr., 42(2), p249-254.
[https://doi.org/10.3746/jkfn.2013.42.2.249]