
Effect of Heat Processing of Royal Jelly at 52 and 72°C on the Growth and Development of Apis mellifera L. Honey Bee Larvae in vitro (Hymenoptera, Apidea)
Abstract
The larval nutritional state of the honey bees is controlled by the Royal Jelly. In addition, it stimulates the regeneration of tissues in other organisms, which has been one of the reasons it uses for cosmetics and as a nutritional ingredient. For the manufacturing and storage of products containing Royal Jelly, heat processing has played a significant role but it has not yet been clarified as a technical factor. The aim of the research was to investigate the effects of heating on the physiological activity of Royal Jelly. We studied the proteins at the native and denatured conditions using heating by the electrophoresis methods. Also, the experiments in vitro were applied when the diets included the non-heated and heated at 52, and 72°C Royal Jelly. The study determined that heating it at 52°C within 5 minutes didn’t change the visible protein composition. This diet was sufficient for the rearing of larvae and hatching of 78.7% against 83.3% of A. mellifera honey bees fed by the diet with non-heated Royal Jelly in the in vitro experiment. However, the weights of the hatched honey bees had significant differences (α=0.05) and were 97.42±14.45 and 102.09±12.49 mg, respectively. This study adds to our understanding of the upper limit of the heat resistance of Royal Jelly proteins by demonstrating the physiological activity of the components after heating at 52°C. So, this temperature can be applied in the protocol of the manufacturing of the Royal Jelly products.
Keywords:
Royal jelly, In vitro, Apis mellifera L., Heat processing, Total protein composition, Honey beeINTRODUCTION
Royal Jelly (RJ) is a natural substance secreted by worker bees to regulate the life of the honey bee colonies. According to the standard ISO 12824, RJ is defined as a mixture of worker bees’ hypopharyngeal and mandibular glands secretions, without any additives. RJ provides the control of the cast determination and the colony development through the larvae’s nutritional state. It was found that the young larvae and queen bees fed from the first to the third day of larvae development by RJ, after which larvae of queen bees feed on RJ, and larvae of worker bees feed on a mix of RJ, honey, and pollen (Weaver, 1957; Page et al., 1995; De Soursa et al., 2018; Maleszka, 2018). Despite the same genetics, the food defines the ontogenesis of larvae as workers or queens with different lifespans and duties in the colony (Kunugi and Ali, 2019). Because the RJ regenerates the cells and tissues of other organisms (Narita et al., 2006; Khazaei et al., 2018), it is an attractive ingredient in health products in both the food and the cosmetic industries (El-Seedi et al., 2022). These properties are provided by the RJ components which include a considerable amount of proteins, free amino acids, lipids, vitamins, sugars, and bioactive substances such as 10-hydroxy-trans-2-decenoic acid, antibacterial protein, and 350-kDa protein (El-Seedi et al., 2022).
To unify the quality of RJ as a product, experts headed by the secretariat of the Standardization Administration of China (SAC, https://www.iso.org/committee/6716626.html) identified the quality of RJ in the Standard ISO 12824 in 2016. However, the full document, especially the details about how to apply some of the methods is not available in open access. In this case, more people can be guided by the Standard methods for Apis mellifera RJ research (Hu et al., 2017), where the methods are described in more detail. So, Lithuanian RJ matched the Standard requirements (Perminaitė et al., 2020), but Saudi RJ corresponded in terms of 10-HDA concentration (Bazeyad et al., 2022). Although RJ collected in one country (Italia) was discriminated by the composition (Mazzei et al., 2020) the quality of their RJ was not compared with the quality declared in Standard. In summary, assessing the quality of RJ by several researchers from different countries is an important step towards unifying the quality of RJ as a product for human health on a global scale.
However, for the manufacturing of products containing RJ, an important technical factor is the temperature of storage and processing, which retains the physiological activity of RJ proteins. Moreover, heat is considered the most familiar denaturing agent for proteins (Acharya and Chaudhuri, 2021). At denaturation, a protein loses its native shape due to the breaking of the weak chemical bonds which makes the protein physiologically inactive (Acharya and Chaudhuri, 2021). Another reaction that leads to a protein alteration by heat processing is the Maillard reaction between carbohydrates and amino acids (Mazzei et al., 2020). Because of this, Sabatini et al. (2009) stored the RJ in a frozen state to prevent the decomposition of biologically active proteins and they recommended that RJ should be frozen as soon as it is harvested. However, the analytic data confirmed that exposure to a temperature of 4℃ caused no alterations in RJ composition (Mazzei et al., 2020). Temperatures that are higher than 8℃ may alter the RJ composition by the Maillard reaction (Mazzei et al., 2020). This reaction was widely studied in dairy products that were stocked in conditions of 70℃ for four days, and the protein concentrate had been altered by at least 67 volatile compounds (Nursten, 1990). So, the Maillard reaction becomes important when heat is applied in manufacturing or for longer storage of the products (Nursten, 1990), including products with RJ which was researched by Hu et al. (2021). They stored the RJ at -20, 4℃, RT, and 37℃ for different times and then studied the conformation changes of proteins. This allowed for clarifying the heat characteristics of protein folding using the spectroscopy method (Rabe et al., 2015). Also, heat resistance was found in Major Royal Jelly Protein 1 (MRJP1) Oligomer (Moriyama et al., 2015). Moreover, the heat characteristic of Apis cerana and Apis mellifera worker bees provides a specific defensive behavior (“hot defensive bee ball”) used against the giant hornet, Vespa mandarinia (Kamioka et al., 2022). Hundreds of worker bees surrounded a hornet and made a “bee ball” where the temperature can reach up to 45-46℃ and it maintains the ball for around 20-30 minutes (Ono et al., 1987; Ken et al., 2005; Kamioka et al., 2022). The wasp surrounded by honey bees perishes at this temperature. So, the heat resistance characteristic of honey bees can be expected at the RJ also, but the influences of high temperatures on the RJ composition in terms of the physiological activity of RJ are not yet studied.
The aim of this study was to investigate the effect of heat processing on the physiological activity of the RJ. In this study, seven temperatures were taken: 32, 42, 52, 62, 72, 82, and 100℃ to the process of RJ by heating it within 5 minutes, and the protein composition in terms of water-solved protein composition was visualized by one-dimensional electrophoresis (1-DE) and two-dimensional electrophoresis (2-DE) methods. Next, the two temperatures (52, and 72℃), at which RJ had distinctive protein fingerprints, were chosen to evaluate the physiological activity by in vitro method. According to our research, the protocol of the manufacturing of the product with RJ may be complemented with regard to the application of the temperatures with the guaranteed preservation of physiological activity RJ components.
MATERIALS AND METHODS
1. Materials
This study was carried out in the Bee breeding Laboratory, Department of Agricultural Biology, National Institute of Agricultural Science (NIAS), the Republic of Korea. Six strong queenright of Apis mellifera ligustica honey bee colonies were kept at the institute apiary and were used for RJ and larvae collection from June to August 2021, and 2022. The RJ of A. m. ligustica honey bees for this research were collected according to recommendations (Hu et al., 2017) and stored at a temperature at -20℃ in the freezer.
2. Sample preparation and analysis of protein content
The samples of RJ, heated RJ, diet with non-heated (fRJ), and heated RJ (fRJ52, and fRJ72) were prepared.
The heated process of previously weighted RJ samples was done in the individual 1.5 mL tubes using Dry Block Heater DB1 (LABTron Co). Seven temperatures were taken with increments of 10 degrees: 32, 42, 52, 62, 72, 82, and 100℃ to the process of RJ by heating within 5 min.
Samples of diets fRJ, heated RJ by 52℃ (fRJ52), and by 72℃ (fRJ72) were mixed by the standard protocol for larvae rearing in vitro (Aupinel et al., 2005). The components of diets are described in Table 1. Samples with 20% of RJ, heated RJ, or diet with RJ were prepared in Tris-HCl 0.025 M buffer with pH=7.4 in ice. Each sample was analyzed at the native (N-sample) and denatured (SDS-sample) conditions of protein structures. Triplicate experiments were run to confirm the reproducibility of the results. The standard protocols of 1-DE (Nowakowski et al., 2014) and 2-DE (Lasserre and Menard, 2012) methods were applied.

Standard larval diet according to Aupinel et al. (2005). Feeding took place over six days. On day one and two, the larvae received 20 μL of diet, on days three to six, larvae received 30 μL, 40 μL, and 50 μL, respectively
Briefly, 1-DE for N-sample preparation, samples were mixed in the ratio of 1 : 3 (v/v) with the native buffer (Sarvari et al., 2022). For SDS-sample preparation, samples were mixed in the ratio of 1 : 3 (v/v) with the Lammli buffer and heated at 82℃ for 5 min for denaturation of protein structure (Schägger, 2006; Kielkopf et al., 2021). After cooling down to room temperature, the same volume of the mixture (10 μL of each sample) was loaded into gels (4% stock, 12% separate gel) (Schägger, 2006). Precision Plus Protein Standard Ladder (Dual color) with molecular weight proteins of 15, 20, 25, 37, 50, 75, 100, 150, and 250 kDa (Bio-Rad) was used for calibration purposes. The gels were then run initially at 20 mA for 30 min, and then the current was increased to 50 mA till the end of the separation. The gels were stained overnight with Coomassie brilliant blue G-250 solution (consisting of one R250 tablet in 2 liters of 10% acetic acid), then were stained with boiled distilled water within 5 minutes for three times, and gels were kept in distilled water at room temperature. The gels were finally visualized by Lightbox (Portable Machine, Iljine D&E).
At 2-DE samples were prepared with 7 M Urea, 2 M Thiourea, 4% CHAPS, and 2.5% DTT buffer. There were 500 μg each sample to the isoelectric focusing with grade pH from 3 to 10, after that the electrophoresis was applied in a Large 12.5% gel (Lasserre and Menard, 2012). The fixing and staining of proteins were provided by the Coomassie brilliant blue G-250 solution.
Digitized 1-DE, and 2-DE gel patterns were edited and matched using Demo program UN-SCAN-IT gel Analysis Software (USA) (https://www.silkscientific.com/demo/), and Delta2D trial version software (DECODON) respectively (https://www.decodon.com/delta2d.html).
3. In vitro rearing
The honey bees were reared in the laboratory under the standard feeding diet as established previously (Aupinel et al., 2005) with the correction of heating the RJ according to the experimental schedule (Table 1). To check the influence of heating on the function activity RJ components, we prepared the diets with RJ (fRJ, fRJ52, and fRJ72). These experimental diets contained the 50% frozen RJ, or after heating at 52, and 72℃ for 5 min respectively. The diets in the 1.5 mL tubes were preserved before using at -4℃ for 4 days, after that we prepared a fresh diet (Toan et al., 2014).
We controlled the age by caging each queen onto an empty comb three days prior to the day of larval emergence as described in research (Hu et al., 2017; Schilcher et al., 2022). Cages had easy access for the worker bees. Upon emergence, the larvae were transported into the lab, individually grafted, and placed into small plastic cups (cups, South Korea). The 48-well plate had 12 cups by 12 larvae for each experimental group; they contained 20 μL of diets which were named N (control), 52, and 72 (Fig. 3A). Well plates with cups, and diets were preheated to 35℃ before grafting. Subsequently, totally 108 larvae of positive control (fRJ), 108 larvae of the experiment (fRJ52), and 108 larvae of expected negative control (fRJ72) were grafted on nine plates according to recommendations (Aupinel et al., 2005). Afterward, the well plates were transferred to an incubator maintained at 35℃ and 95% relative humidity (RH). Larvae were fed over six consecutive days according to Table 1.
Once the larvae transformed into pupae, they were transferred into fresh 24-well plates with 2 layers of UV-cleaned dust-free Kimwipes appropriate size (Toan et al., 2014), transferred into the same incubator and left untouched, apart from sparse mortality check-ups, until emergence. After that, the active honey bees that also had a dark color were weighed on the scales.
4. Statistical analysis
The data was analyzed by Descriptive Statistics to characterize the groups, by One-way ANOVA followed by Tukey’s post hoc test for comparing three and more groups, and by t-test to compare two groups, where the significant levels were set at α=0.05. The SAS Enterprise Guide 7.1 statistical software (ver. 25), MS Excel with the XLSTAT, AtteStat application, and program R were used for statistical calculations and visualization of data.
RESULTS
1. The upper limit of heat resistance of water-soluble proteins of Royal Jelly
Prior to the choice of the experimental temperatures, the heated process of RJ samples was done at seven temperatures 32, 42, 52, 62, 72, 82, and 100℃. The samples after heating were compared against the control sample (non-heated RJ) by the 1-DE method and model gel (Fig. 1A).
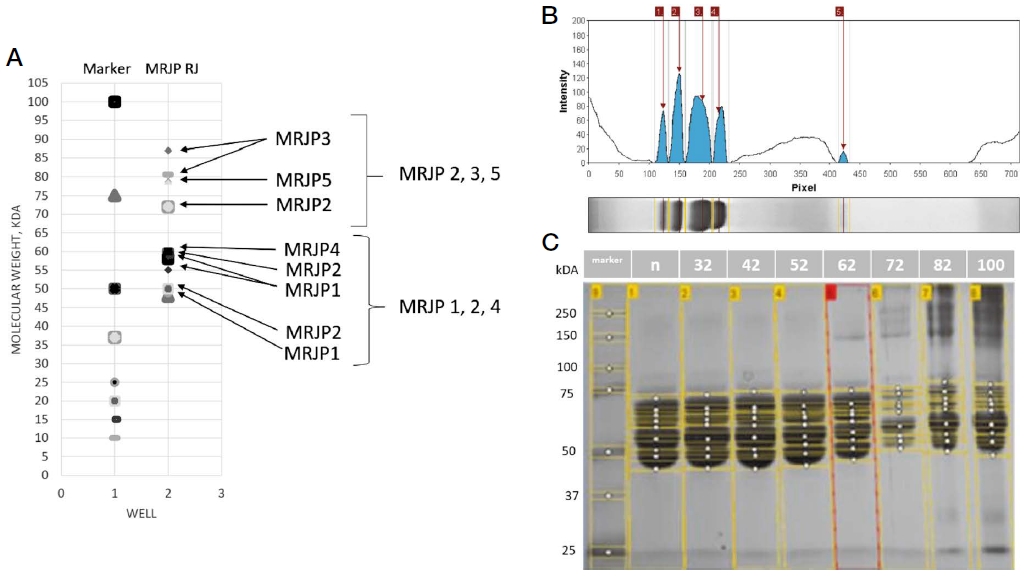
The evaluation of heat processing results of the Royal Jelly samples. A - Model band profiles of RJ proteins by 1-DE electrophoresis. The molecular masses of the main RJ proteins were taken from the NCBI database. B - The numeric estimation and visualization of protein bands on the gel (one well) by the UN-SCAN-IT gel Analysis Software. C - The visualization of the water-soluble proteins by 1-DE electrophoresis. Each well contains a sample of RJ after heat processing: non, 32, 42, 52, 62, 72, 82, and 100℃, respectively.
We found, that the fingerprints of the proteins with heated RJ up to 52℃ had no significant differences against the control sample (one-way ANOVA, Tukey post hoc test) (Fig. 1C), and what we have concluded about the heat resistance of WSPs. Next heating of RJ samples (62, 72, 82, and 100℃) lead to the protein’s degradations (Fig. 1C). Moreover, the heat resistance of proteins at 52℃ was confirmed by 2-DE method. On this basis, temperatures of 52, and 72℃ were chosen for next testing WSPs in the diets for in vitro experiment.
Next, to check the WSPs in diets by the 1-DE method, we prepared the diets with RJ (fRJ, fRJ52, and fRJ72). The WSPs in each sample was tested in native (N) and denatured (SDS) conditions (Fig. 2). The same fingerprints of WSPs in fRJ52, and fRJ samples were confirmed, and the proteins in fRJ72 sample were not visualized (Fig. 2).
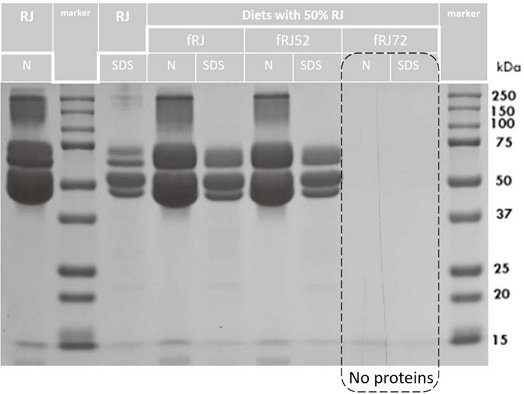
Fingerprint 1-DE of the larvae diets with RJ after heat processing at 52, and 72℃ for 5 min (fRJ52, and fRJ72). N - native condition of water-soluble proteins (WSPs) in the sample; SDS - denature condition of WSPs in the sample.
So, we visualized the similar fingerprints of the WSPs samples RJ heated at 32, 42, and 52℃, weaker visualization of WSPs after heating of RJ at 62, and smooth visualization after heating of RJ at 72℃ for 5 min. Some WSPs were obviously heat resistant, whereas others were lost after heating at 62℃ and higher. Moreover, we didn’t visualize the WSPs in the diet fRJ72. On this basis, we suggested the upper limit of heat resistance of WSPs of Royal Jelly up to 52℃ and it was tested in next experiment below.
2. The test of physiological functions of RJ in vitro
To check the influence of heating at 52, and 72℃ on the physiological function of the RJ components, we applied in vitro larvae rearing. In the larvae stage, each plate contains the same number of larvae fed by three different diets: RJ (fRJ control), heated RJ by 52℃ (fRJ52, experiment), and by 72℃ (fRJ72, negative control) (Fig. 3A-C). The survival rate of the larvae (pupae) fed fRJ, fRJ52, and fRJ72 were 100 (83)%, 98 (80)%, and 4 (0)%, respectively. The emergence rates of honey bees were 84.91%, 78.70%, and 0% for the fRJ, fRJ52, and fRJ72 diets, respectively (Fig. 4A).
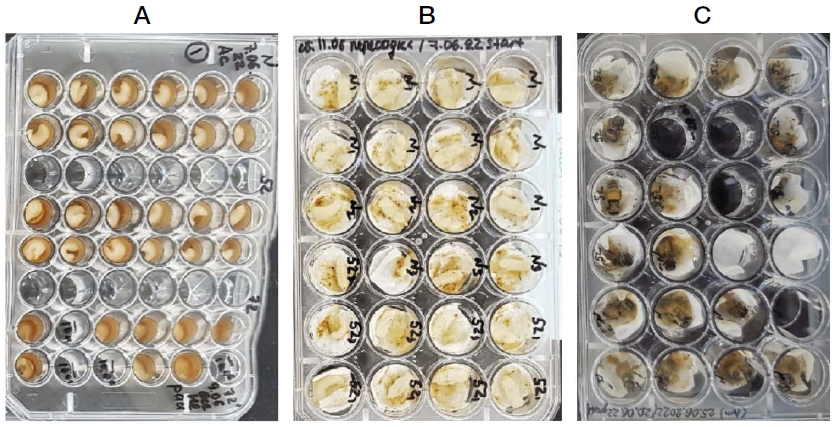
The stages of rearing Apis mellifera worker bees with different diets (the temperature of heat processing of RJ: non, 52, and 72℃) in vitro: A - larvae stage; B - pupae stage; C - hatching honey bees.
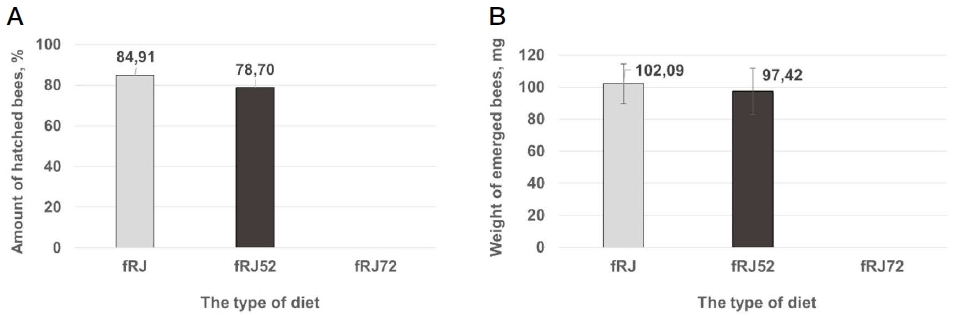
The influence of diet using the heat processing of the RJ on the metamorphosis of A. mellifera honey bees in vitro: A - The number of hatched honey bees on 15-16 days of development, %; B - The weight of 1-day alive hatched honey bees, mg.
Hatched in vitro honey bees fed fRJ had a weight of 102.09±12.49 mg (n=74). In contrast, honey bees fed fRJ52 were significantly lighter by weight (97.42±14.45 mg; n=74) than the honey bees fed fRJ (t-test: t=2.091; df=140; P<0.05). Acute larvae mortality by consuming the heated fRJ72 diet was indicated, so the modification of RJ components at the heating was a significant factor to depress the development of larvae.
DISCUSSION
In this study, the effects of the heated process of RJ were estimated by the amounts of WSPs at heating temperatures up to 32, 42, 52, 62, 72, 82, and 100℃ at the native (N-sample) and denatured (SDS-sample) conditions of protein structures by electrophoresis methods. Then, two distinctive temperatures (52, and 72℃) were chosen to evaluate the physiological functions of RJ components by observing the growth and development of Apis mellifera L. honey bee larvae in vitro.
The denatured (SDS-sample) condition is a biological phenomenon in which a protein loses its native shape, which makes the protein biologically inactive (Acharya and Chaudhuri, 2021). In this case, we controlled the protein composition of RJ by comparing of the native and denatured conditions. The test of the native state of the sample excludes the loss of small proteins in low concentrations that are non-visible after the denaturing process (Nowakowski et al., 2014). On the other hand, the globular proteins in their native state may be lost because they can be locked in the gel because of the protein complex structure. So, both the N and SDS states of WSPs were visualized to evaluate the total protein composition of RJ (Fig. 1C, 2). Moreover, Biam et al. (2022) reported that sample heating drastically affected the ability to detect proteins following separation by SDS-PAGE. However, we discovered identical visualizations of WSPs in N and SDS states, demonstrating minor differences in molecular mass of WSPs before and after denaturation. It demonstrated the peculiarity of the protein structure RJ, which could influence heat resistance and should be investigated further in the future.
The temperature at which proteins begin to degrade was also investigated. Some researchers reported that the A. cerana honey bee’s lethal temperature is 50-51℃ (Ken et al., 2005); that is, at this temperature, the beginning of protein destruction inside the alive whole organism begins, and we expected protein degradation to begin when the RJ samples were heated to 52℃. However, we found stable visualization of the WSPs samples when RJ was heated at 52℃. Therefore, we carried out the physiological test in the in vitro experiment of heated RJ at 52℃ as upper limit of heat resistance of WSPs of RJ. Our results confirmed not only the heat resistance of structure WSPs RJ at 52℃ but stability physiological function also. Moreover, we visualized the beginning destruction of low-weight WSP RJ at 62℃, but it was not the MRJP1 location. The heat resistance of MRJP1 forms was reported by Moriyama et al. (2015) and they found converted to macromolecular heat-aggregated MRJP1 oligomer at 65℃. Also, the first inflection temperature of protein structures of the RJ was 64.6℃ (Hu et al., 2021). We confirm these outputs by the successful rearing of honey bees in vitro fed by a diet containing the RJ heating at 52℃. The tested temperature was less than the reported 64.6℃ when the disturbance of the structure of RJ proteins was started. It proves the presence of the undamaged components of RJ in the diet. However, the heating at 52℃ significantly decreased the weight of hatched adult honey bees compared to control honey bees. Using these conclusions, we cannot recommend the application of the pasteurization methods in the processing of the RJ contain products because the temperature of pasteurization methods starts from 63℃ (Robinson, 2002; Atia et al., 2011).
The second inflection temperature of the fresh RJ and the RJ samples stored at -80℃ was 72.6℃ (Hu et al., 2021). At this temperature, we detected the impossibility of the larvae’s growth and transformation into pupae. Additionally, the RJ proteins were not visualized successfully in the diet. Therefore, this conclusion was confirmed in our research by the disturbance of proteins and physiology functions caused by heating RJ at 72℃ for 5 min.
In this investigation, we did not examine additional RJ components that had been heated in addition to proteins. However, we must acknowledge that every single RJ component, without exclusion, affects in vitro larval development. The temperatures were entirely chosen based on WSP data.
CONCLUSION
In this study, we estimated the effects of heating up to 32, 42, 52, 62, 72, 82, and 100℃ by the visualization of RJ water-soluble proteins and chose 52 and 72℃ with clear and smooth visualization, respectively, against non-heated RJ to check the physiology activity of RJ components by the in vitro larvae rearing method. We found that the diet with Royal Jelly heated at 52℃ within 5 minutes was sufficient for the rearing of larvae and hatching of 78.7% versus 83.3% of A. mellifera honey bees fed by the diet with non-heated Royal Jelly. However, the weights of the hatched honey bees had significant differences (α=0.05) and were 97.42±14.45 and 102.09±12.49 mg, respectively. This study adds to our understanding of Royal Jelly’s heat resistance by demonstrating that the physiological activity of the components was not destroyed when heated at 52℃ for 5 min. So, we recommend this temperature as the upper limit of the heat resistance of WSPs of Royal Jelly. However, we cannot recommend the application of the pasteurization methods in the processing of the RJ-containing products because the temperature of the pasteurization methods starts at 63℃. But, in the protocol of the manufacturing of the RJ products can apply the heating up to 52℃.
Acknowledgments
We gratefully thank the bee breeding laboratory, especially Kim Ho Jin, the National Institute of Agricultural Science (NIAS), and the Rural Development Administration (RDA). We wish to thank Dr. Sovetgul Asekova, Dr. Ural Yunusbaev, Dr. Alexandr Vasilievich Petuchov, and Karla Cristina Valdovinos for their valuable discussions. This research was funded by a grant from the Research Program for Agricultural Science & Technology Development (Project No. PJ01575503), National Institute of Agricultural Sciences, Rural Development Administration, Republic of Korea.
References
-
Acharya, V. V. and P. Chaudhuri. 2021. Modalities of Protein Denaturation and Nature of Denaturants. Int. J. Pharm. Sci. Rev. Res. 69(2): 19-24.
[https://doi.org/10.47583/ijpsrr.2021.v69i02.002]
- Atia, F. M., M. M. Mostafa, M. A. El-Nono and M. F. Abdel-Salam. 2011. Solar Energy Utilization for Milk Pasteurization. Misr J. Ag. Eng. 10(28): 729-744.
- Aupinel, P., D. Fortini, H. Dufour, J. N. Tasei, B. Michaud, J.-F. Odoux and M.-H. Pham-Delègue. 2005. Improvement of artificial feeding in a standard in vitro method for rearing Apis mellifera larvae. Bull. Insectology 58: 107-111.
-
Bazeyad, A. Y., A. A. Al-Ghamdi and Y. Z. Alattal. 2022. Physicochemical characteristics of local royal jelly produced in Al-Baha region, Saudi Arabia. N.a. J. Adv. Res. Rev. 14(01): 284-292.
[https://doi.org/10.30574/wjarr.2022.14.1.0327]
-
Biam, R. S., P. P. Robichaud, M. Mbarik, P. Pineau and M. E. Surette. 2022. Loss of detection of fatty acid-metabolizing proteins in Western blot analyses - Impact of sample heating. Biochem. Biophys. Res. Commun. 607: 110-116.
[https://doi.org/10.1016/j.bbrc.2022.03.130]
-
De Souza, D. A., M. H. Huang and D. R. Tarpy. 2018. Experimental improvement of honey bee (Apis mellifera) queen quality through nutritional and hormonal supplmentation. Apidologie 50: 14-27.
[https://doi.org/10.1007/s13592-018-0614-y]
-
El-Seedi, H. R., N. Eid, A. A. Abd El-Wahed, M. E. Rateb, H. S. Afifi, A. F. Algethami, C. Zhao, Y. Al Naggar, S. M. Alsharif, H. E. Tahir, B. Xu, K. Wang and S. A. M. Khalifa. 2022. Honey Bee Products: Preclinical and Clinical Studies of Their Anti-inflammatory and Immunomodulatory Properties. Front. Nutr. 3(8): 761267.
[https://doi.org/10.3389/fnut.2021.761267]
-
Hu, F.-L., K. Bılikova, H. Casabianca, D. Gaelle, F. S. Espindola, M. Feng, C. Guan, B. Han, T. K. Krakova, J. K. Li, L. Li, X. A. Li, J. Simuth, L. M. Wu, Y. Q. Wu, X. F. Xue, Y. B. Xue, K. Yamaguchi, Z. J. Zeng, H. Q. Zheng and J. H. Zhou. 2017. Standard methods for Apis mellifera Royal Jelly research. J. Apic. Res. 56: 1-68.
[https://doi.org/10.1080/00218839.2017.1286003]
-
Hu, H., Q. Wei, Z. S. X. Zhang, C. Ma, M. Feng, L. Meng, J. Li and B. Han. 2021. Development of a Freshness Assay for Royal Jelly Based on the Temperature- and Time-Dependent Changes of Antimicrobial Effectiveness and Proteome Dynamics of Royal Jelly Proteins. J. Agric. Food Chem. 69(36): 10731-10740.
[https://doi.org/10.1021/acs.jafc.1c02843]
- International Standard Organization. 2016. ISO 12824 standard «Royal Jelly - Specifications». First edition.
-
Kamioka, T., H. C. Suzuki, A. Ugajin, Y. Yamaguchi, M. Nishimura, T. Sasaki, M. Ono and M. Kawata. 2022. Genes associated with hot defensive bee ball in the Japanese honeybee, Apis cerana japonica. BMC Ecol. Evol. 22(1): 31.
[https://doi.org/10.1186/s12862-022-01989-9]
-
Ken, T., H. R. Hepburn, S. E. Radloff, Y. Yusheng, L. Yiqiu, Z. Danyin and P. Neumann. 2005. Heat-balling wasps by honeybees. Naturwissenschaften 92: 492-495.
[https://doi.org/10.1007/s00114-005-0026-5]
-
Khazaei, M., A. Ansarian and E. Ghanbari. 2018. New Findings on Biological Actions and Clinical Applications of Royal Jelly: A Review. J. Diet. Suppl. 15(5): 757-775.
[https://doi.org/10.1080/19390211.2017.1363843]
-
Kielkopf, C. L., W. Bauer and I. L. Urbatsch. 2021. Sodium Dodecyl Sulfate-Polyacrylamide Gel Electrophoresis of Proteins. Cold Spring Harb. Protoc. 2021.
[https://doi.org/10.1101/pdb.prot102228]
-
Kunugi, H. and M. A. Ali. 2019. Royal Jelly and Its Components Promote Healthy Aging and Longevity: From Animal Models to Humans. Int. J. Mol. Sci. 20(19): 4662.
[https://doi.org/10.3390/ijms20194662]
-
Lasserre, J. P. and A. Menard. 2012. Two-dimensional blue native/SDS gel electrophoresis of multiprotein complexes. Methods Mol. Biol. 869: 317-337.
[https://doi.org/10.1007/978-1-61779-821-4_27]
-
Maleszka, R. 2018. Beyond Royalactin and a master inducer explanation of phenotypic plasticity in honey bees. Commun. Biol. 1: 8.
[https://doi.org/10.1038/s42003-017-0004-4]
-
Mazzei, P., A. Piccolo, M. Brescia and E. Caprio. 2020. Assessment of geographical origin and production period of royal jelly by NMR metabolomics Chem. Biol. Technol. Agric. 7: 24.
[https://doi.org/10.1186/s40538-020-00190-8]
-
Moriyama, T., A. Ito, S. Omote, Y. Miura and H. Tsumoto. 2015. Heat Resistant Characteristics of Major Royal Jelly Protein 1 (MRJP1) Oligomer. PLoS ONE 10(5): e0119169.
[https://doi.org/10.1371/journal.pone.0119169]
-
Narita, Y., J. Nomura, S. Ohta, Y. Inoh, K. M. Suzuki, Y. Araki, S. Okada, I. Matsumoto, Y. Isohama, K. Abe, T. Miyata and S. Mishima. 2006. Royal jelly stimulates bone formation: physiologic and nutrigenomic studies with mice and cell lines. Biosci. Biotechnol. Biochem. 70(10): 2508-2514.
[https://doi.org/10.1271/bbb.60240]
-
Nowakowski, A. B., W. J. Wobig and D. H. Petering. 2014. Native SDS-PAGE: high-resolution electrophoretic separation of proteins with retention of native properties including bound metal ions. Metallomics 6(5): 1068-1078.
[https://doi.org/10.1039/C4MT00033A]
- Nursten, H. E. 1990. Key mechanistic problems posed by the Maillard reaction. pp. 145-153. in The Maillard Reaction in Food Processing, Human Nutrition and Physiology, eds. by P. A. Finot, H. U. Aeschbacher, R. F. Hurrell and R. Liardon. Birkhäuser, Basel.
-
Ono, M., I. Okada and M. Sasaki. 1987. Heat production by balling in the Japanese honeybee, Apis cerana japonica as a defensive behavior against the hornet, Vespa simillima xanthoptera (Hymenoptera: Vespidae). Experientia 43: 1031-1034.
[https://doi.org/10.1007/BF01952231]
-
Page, R. E., K. D. Waddington, G. J. Hunt and M. K. Fondrk. 1995. Genetic determinants of honey bee foraging behavior. Anim. Behav. 50: 1617-1625.
[https://doi.org/10.1016/0003-3472(95)80015-8]
-
Perminaite, K., M. Marksa, L. Ivanauskas, A. M. Inkeniene, A. Grigonis and K. Ramanauskiene. 2020. Lithuanian Royal Jelly: quality assessment, biological activity and quantitative determination of trans-10-hydroxy-2-decenoic acid in various solvents. Chemija. 31(3): 162-169.
[https://doi.org/10.6001/chemija.v31i3.4290]
-
Rabe, M., A. Boyle, H. R. Zope, F. Versluis and A. Kros. 2015. Determination of oligomeric states of peptide complexes using thermal unfolding curves. Biopolymers 104(2): 65-72.
[https://doi.org/10.1002/bip.22598]
- Robinson, K. 2002. Changes to Milk Components During Processing. pp. 31-35. in Dairy microbiology handbook. 765 p. Wiley and Sons Inc., New York, USA.
- Sabatini, A. G., G. L. Marcazzan, M. F. Caboni, S. Bogdanov and L. B. de Almeida-Muradian. 2009. Quality and standardization of Royal Jelly. JAAS 1(1): 1-6.
-
Sarvari, E., G. Gellen, M. Sagi-Kazar, G. Schlosser, K. Solymosi and A. Solti. 2022. Qualitative and quantitative evaluation of thylakoid complexes separated by Blue Native PAGE. Plant Methods 18(1): 23.
[https://doi.org/10.1186/s13007-022-00858-2]
-
Schägger, H. 2006. Tricine-SDS-PAGE. Nat. Protoc. 1: 16-22.
[https://doi.org/10.1038/nprot.2006.4]
-
Schilcher, F., L. Hilsmann, L. Rauscher, L. Degirmenci, M. Krischke, B. Krischke, M. Ankenbrand, B. Rutschmann, M. J. Mueller, I. Steffan-Dewenter and R. Scheiner. 2022. In Vitro Rearing Changes Social Task Performance and Physiology in Honeybees. Insects 13: 4.
[https://doi.org/10.3390/insects13010004]
-
Toan, T. V., M.-L. Lee, H.-S. Sim, H.-K. Kim, G.-H. Byuon and Y.-S. Choi. 2014. Initial Results of Rearing Honey bee Apis cerana in vitro. J. Apic. 29: 193-197.
[https://doi.org/10.17519/apiculture.2014.09.29.3.193]
-
Weaver, N. 1957. Effects of Larval Age on Dimorphic Differentiation of the Female Honey Bee. Ann. Entomol. Soc. Am. 50: 283-294.
[https://doi.org/10.1093/aesa/50.3.283]
- Trial version Delta2D (DECODON) (https://www.decodon.com/delta2d.html, ).
- UN-SCAN-IT gel Analysis Software (USA) (https://www.silkscientific.com/demo/, ).