
Using Image Deep Learning to Measure Flight Speeds and Patterns of Honeybees (Apis mellifera) and Bumblebees (Bombus terrestris) near Their Hives
Abstract
Bee traffic at the hive entrance can be used as an important indicator of foraging activity. We investigated the flight speed and patterns of honeybees and bumblebees near their hives as a basis for calculating bee traffic using the image deep learning. The flying speed of bumblebees (0.48±0.36 m/s) near the hive was 1.4 times faster than that of honeybees (0.35±0.21 m/s). The flight speed of honeybee leaving the hive (0.54±0.33 m/s) was 1.7 times faster than that when entering the beehive (0.32±0.18 m/s). Distance from the hive and flight speed showed a positive correlation (honeybee r=0.600, bumblebee 0.659), and a significant linear regression model was derived (honeybee R2=0.516, bumblebee 0.433). The flight pattern near the hive differed significantly according to bee at entering and leaving the hive. Honeybees mainly showed flight that changed flight direction more than once (69.5%), whereas bumblebees mainly performed straight flight (48.7%) or had a single turn (36.5%) in flight. When bees entered the hive, honeybees primarily showed one-turn or two-turn flight patterns (88.5%), and bumblebees showed a one-turn flight pattern (48.0%). In contrast, when leaving the hive, honeybees primarily showed a straight flight pattern (63.0%), and bumblebees primarily showed a straight or one-turn pattern (90.5%). There was a significant difference in flight speed according to the flight pattern. The speed of straight flight (0.89±0.47 m/s) was 1.5 to 2.1 times faster than flight where direction changed. In summary, the speed and pattern of bees returning to or leaving the hive were different to from to the hive, and there were also differences between bee species. Therefore, our results can help determine the ideal frame rate for effectively capturing and recognizing the flying image of bees when calculating bee traffic by image deep learning.
Keywords:
Flight pattern, Flight speed, Honeybee, Bumblebee, Deep learningINTRODUCTION
Bees (Hymenoptera: Apidae) play a crucial role in the pollination of plants consumed as food by humans. The pollination service provided by bees contributes to 35% of total crop production, and 87% of the leading food crops, across the whole world (Klein et al., 2007; Gallai et al., 2009). Honeybees and bumblebees are the most commonly used commercial pollinators worldwide, and used in orchard crops such as almonds and apples, and greenhouse crops such as tomatoes and strawberries (Abak et al., 1995; Velthuis and van Doorn, 2006).
Bee traffic, which is defined as the number of bees leaving and entering an area, can directly identify bee activity, and it can be used as an important indicator of colony health, colony age, climate change, honey flow, and foraging activity (Gary, 1992; Meikle and Holst, 2015). Various techniques for automatically measuring bee traffic have been attempted, such as infrared sensors (Liu et al., 1990; Struye et al., 1994), analyzing images or videos through cameras (Kulyukin and Mukherjee, 2019; Sun and Gaydecki, 2021), analyzing RFID (radiofrequency identification) tags (Klein et al., 2019; Alburaki et al., 2021; Costa et al., 2021), and radar transponders (Woodgate et al., 2016), because it is difficult to visually inspect the activity of tens to hundreds of different bees entering and exiting hives. Recently, video image analysis employing smart cameras using deep learning technology has facilitated the detection of bees through images of bee traffic. This method using image deep learning utilizes technology that tracks the flight path of bees by learning images of bees (Dell et al., 2014; Boenisch et al., 2018; Sun and Gaydecki, 2021), but it is difficult to commercialize because it requires the use of very expensive equipment.
In contrast, the method that measures bee traffic in front of a beehive by tracking a specific bee in real time from an image taken by a smart camera, based on deep learning, is very economical, because it is possible using only a webcam and the processing power of a mobile CPU (Lee et al., 2020). This method requires data on the flight speeds and patterns of bees around the beehive to effectively take an image.
Several studies have been conducted previously on the flight speed and flight patterns of bees and bumblebees, the flight physiological and behavioral characteristics of bee pollinators (Baird and Dacke, 2012; Ravi et al., 2019), their adaptability to the environment (Seidl et al., 2017; Lecoeur et al., 2019), or flight kinematics (Crall et al., 2017). However, few studies have quantitatively measured how the flight speeds or patterns change around the beehive to measure bee traffic, particularly using image deep learning. In this study, we investigated the flight speeds and patterns of honeybees and bumblebees near their hives to develop a bee traffic measurement machine that can measure the activity of bees at an affordable price.
MATERIALS AND METHODS
1. Study site and insects
Experiments to investigate the flight speeds and patterns of honeybees and bumblebees near their hives were carried out from July 3 to 5, 2021, in an experimental field at the National Institute of Agricultural Science, Wanju, South Korea (35°49′43″N, 127°02′33″E). To compare the flight speeds and patterns between bee species, a cross between three strains of European honeybee colonies (Apis mellifera L., five frames, over 10,000 workers, styrofoam beehive) selected by the Rural Development Administration, and bumblebee colonies (Bombus terrestris L., 100 workers, plastic beehive) of the 19th generation, reared under artificial conditions (26℃, relative humidity 80%; Yoon et al., 2010), were installed at each of the test sites.
Video recordings of the flying bees were performed daily for an hour, from 3 pm to 4 pm, during the investigation period. In consideration of the effects of weather on changes in bee activity, a weather condition data logger (Illuminance UV recorder TR-74Ui; T&D Co., Matsumoto, Nagano, Japan) was installed on each beehive cover. The temperature, illumination, humidity, and ultraviolet radiation inside the experimental field during the irradiation period were recorded every 10 min.
2. Video recordings of bee flight
To record the flight images of bees, a honeybee hive and a bumblebee hive were installed on two test plots, respectively. To reduce accidental visits by bees to different hives, each test plot was placed 50 m apart. A 50 cm plastic ruler was attached to the beehive lid to serve as a scale to measure the distance from the hive entrance. A camera tripod was installed at a height of 1 m at a distance of 0.3 m from each hive. An iPhone X (Apple Inc.; Cupertino, California, United States) used as the camera for video recording was installed on each tripod, and the image was taken from the top to the bottom (Fig. 1A). Video was recorded five times in “Slo-mo” mode, at 240 fps and in 1,080p mode, for 1 hour, with each video lasting 10 minutes, and simultaneously with two test plots at the same time.
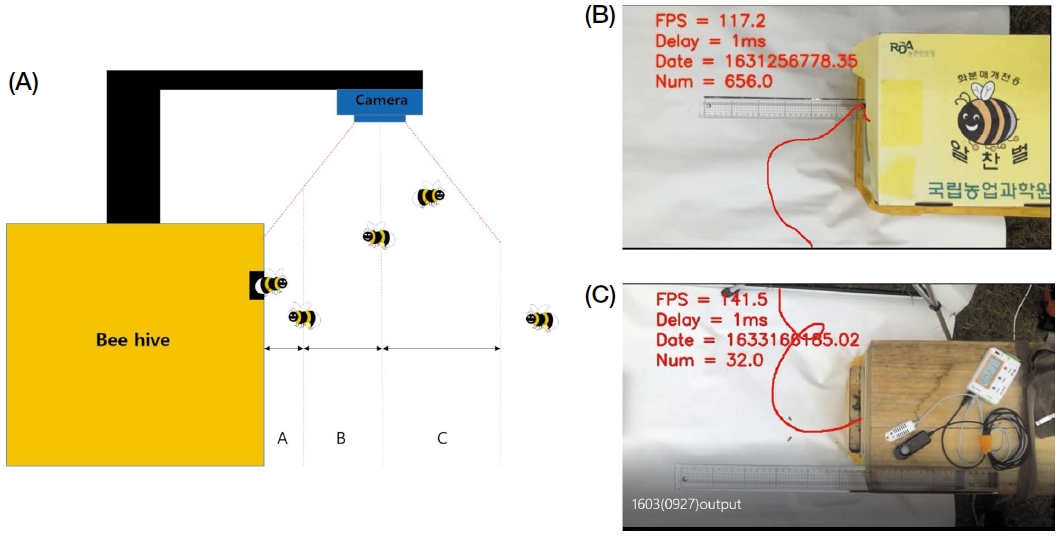
Camera settings in this study. (A) Schematic representation of camera setting in vertical view. A is the area from the entrance of the beehive to 5 cm, B is the area from 5 to 15 cm, and C is the area from 15 to 30 cm; (B) Bumblebee camera settings in top view; (C) Honeybee camera settings in top view. The red solid lines are the flight paths of the bees.
3. Image analysis
Each frame of the captured video was imaged, and then each image was combined through a self-made application program to make a video again, with the frame number inserted and displayed on the video. First, the video was played with a video player (Potplayer; Kakao Corp., Jeju-si, Jeju, Korea); secondly, the image processing program Image J (National Institute of Health, Bethesda, Maryland, United States) was executed; and thirdly, the Image J program was made transparent through a program (Transparent window for w2k, Dog& Cow, Seoul, Korea) that made the application window transparent, after which the flight path of bees was drawn.
The drawn flight path was converted to distance (m), using the ruler as a scale. To calculate the flight speed, the change value of the frames until the flight path was drawn was checked (Δ frame number=number of frames that completed the flight path-number of frames that started the flight path), and ‘Δ frame number’ was divided by 240 to calculate the time (seconds). Flight patterns were classified into four categories (Table 1).
The flight speed and pattern of bees leaving the beehive and returning bees were investigated. In order to investigate the flight speed and flight pattern by distance from the beehive, areas of 5 cm, 15 cm, and 30 cm from the beehive entrance were set.
4. Statistical analysis
We evaluated differences in the flight speed of honeybees and bumblebees leaving and returning to the hive using an unpaired t-test. The effect of distance from the hive on flight speed was tested using a one-way ANOVA test, with a Tukey HSD post-test. The correlation between the distance from the beehive and the flight speed of each bee species was analyzed by Pearson correlation, and a linear regression equation and significance value were derived. Differences in the flight patterns between bee species or bee behaviors were verified by the chi-square test. The difference in flight speed between each flight pattern was verified for significance by one-way ANOVA test and Tukey HSD post-test. In each flight pattern, the difference in flight speed between bee species, and the difference in flight speed between bee behaviors, was evaluated using an unpaired t-test. All statistical analyses were performed using the SPSS PASW 22.0 package for Windows (IBM; Chicago, IL, USA).
RESULTS
1. The flying speed of bees nearby the hive
The flying speed of bumblebees (0.48±0.36 m/s) within 30 cm of the hive was 1.4 times faster than that of honeybees (0.35±0.21 m/s; Fig. 2A; t-test t137= -2.714, p=0.008). In honeybees, the flight speed leaving the beehive (0.54±0.33 m/s) was significantly faster (1.7 times) than when approaching (0.32±0.18 m/s) (Fig. 2B; t87= -3.182, p=0.002), but there was no significant difference in the bumblebee (Fig. 2C). When the bees entered the hive, the bumblebee’s flight speed (0.54±0.35 m/s) was significantly faster than that of the honeybee (0.32±0.18 m/s), by 1.7 times (Fig. 2D, t105= -4.090, p=0.0001), but honeybees (0.54±0.33 m/s) flew 1.3 times faster than bumblebees (0.41±0.37 m/s) when leaving their hives (Fig. 2E, p>0.05).
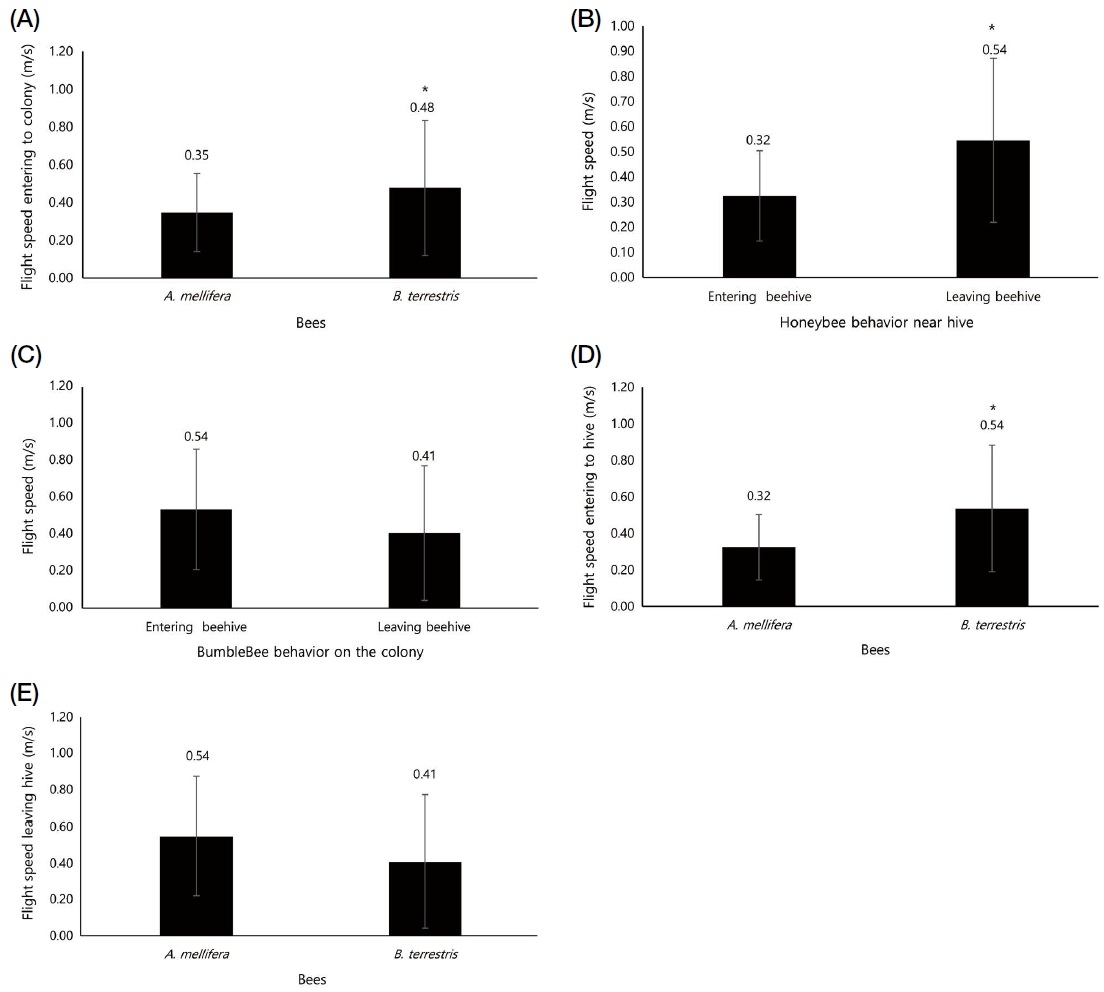
Comparison of flying speeds between Apis mellifera and Bombus terrestris bees near the hive. (A) Comparison of flight speed between honeybees and bees; (B) Flight speeds in relation to honeybee behavior; (C) Comparison of flight speeds by behavior in bees; (D) Flight speeds of bees when entering the hive; (E) Flight speeds of bees when leaving the hive. Error bars are standard deviation values. * indicates that the data were significantly different according to a t-test (p<0.05).
2. Bee flight speed according to distance from hive
Distance from the hive and flight speed showed a positive correlation (Table 2), and a significant linear regression equation was derived (A. mellifera - regression model ANOVA test F1,87=92.866, p=0.0001, DW=1.609; B. terrestris - F1,48=36.654, p=0.0001, DW=1.707; Fig. 3).
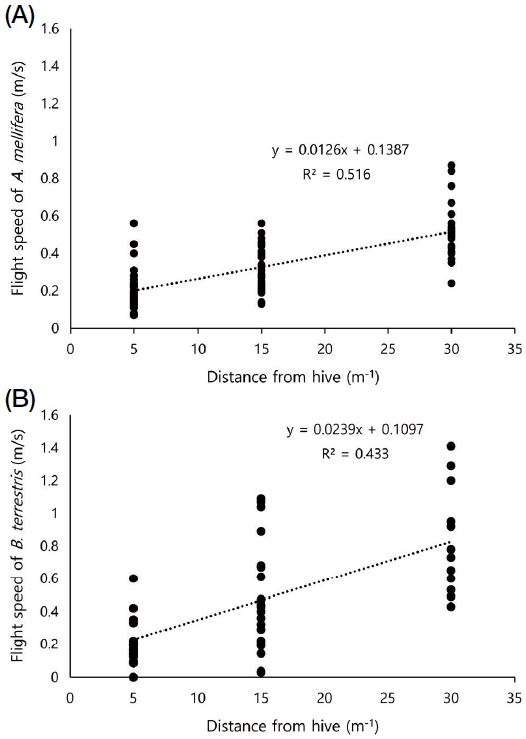
Correlation between flight speeds of Apis mellifera (A) and Bombus terrestris (B) and distance from beehive.
Honeybees and bumblebees showed different flight speeds according to the distance from the hive, respectively (one-way ANOVA test, A. mellifera - F2,86=45.957, p=0.0001, B. terrestris - F2,47=18.347, p=0.0001; Table 3). The honeybee showed flight speeds of 0.20 m/s, 0.36 m/s and 0.52 m/s at 5 cm, 15 cm, and 30 cm from the hive, respectively, and the bumblebee had speeds of 0.21 m/s, 0.50 m/s and 0.81 m/s, respectively. The flight speed was not significantly different between honeybees and bumblebees in the area less than 5 cm from the beehive, but the bumblebee’s flight speed was 1.6 times and faster than that of the honeybee in the area at a distance of 5 cm to 30 cm from the hive (t-test 5-15 cm - t49= -2.852, p=0.006; 15-30 cm - t35= -3.732, p=0.001; Table 3).
The flight speed of honeybees entering the hive decreased by 40% from 30 cm to 15 cm from the hive, and by another 45% from 15 cm to 5 cm (one-way ANOVA test F2,77=61.114, p=0.0001; Table 4). The flight speed of bees leaving the hive increased 1.1 times from 5 cm to 15 cm from the hive (Table 4). The speed when leaving the hive was 2.3 times faster than the speed at entry in the area less than 5 cm from the hive (t-test: t31= -5.885, p=0.0001). There was no significant difference between the behaviors of the honeybees in the area from 5 cm to 15 cm.
The flight speed of bumblebees entering the hive decreased by 11% from 30 cm to 15 cm from the hive, and by another 70% from 15 cm to 5 cm (one-way ANOVA test F2,24=14.931, p=0.0001; Table 4). The flight speed of bumblebees leaving the hive increased 1.1 times and 3.5 time from 5 cm to 15 cm from the hive and from 15 cm to 30 cm from the hive, respectively (F2,20=15.748, p=0.0001; Table 4). There was no significant difference between the behaviors of the bees in the area less than 5 cm from the hive. The speed when entering the hive was 2.9 times faster than the speed at leaving in the area from 5 cm to 15 cm (t-test: t31= -5.885, p=0.0001). There was no significant difference between the behaviors of the bumblebees in the area from 5 cm to 15 cm.
3. Flight patterns of bees nearby the hive
When the flight patterns of bees nearby the hive were divided into four categories (one turn, two turns, circle, straight line; Table 1), one turn and a straight line were the most frequently encountered flight patterns, with more than 70%, while the circular flight was the least used (Table 5). The flight pattern showed a significant difference according to bee species (chi-square test: χ23=26.283, p=0.0001). Honeybees mainly displayed flight that changed flight direction more than once (69.5%), while bumblebees mainly performed straight flight (48.7%) or one turn (36.5%) flight. The bees showed different flight patterns when entering or leaving the hive (χ23=59.945, p=0.0001; Table 5). When honeybees entered the hive, they showed a flight pattern of one or two turns, and when leaving the hive, they mostly showed a straight flight pattern (63%) (χ23=62.231, p=0.0001; Table 6). In contrast, when entering the hive, bumblebees mainly showed a one-turn flight pattern, and when leaving the hive, mostly showed a one-turn (47.6%) or straight flight pattern (42.9%) (χ23=9.095, p=0.028; Table 6).
4. Bee flight speed in flight patterns
In honeybees, the change of direction and the circular flight pattern were approximately the same, at 0.35-0.40 m/s, but the straight flight pattern was 0.90 m/s, which was 2.5 times faster than the other flight patterns (F3,137=55.849, p=0.0001; Fig. 4). In contrast, bumblebees showed no significant differences in flight speeds according to flight pattern (F3,111=0.448, p=0.719; Fig. 4). Even with similar flight patterns, the flight speeds of honeybees and bumblebees were significantly different. In one turn, two turns, and circular flight patterns, the bumblebee was 2.1 times, 2.3 times, and 1.7 times faster than the honeybees, respectively (t-test: one turn t91= -4.840, p=0.0001; two turns t58= -6.565, p=0.0001; circular t12= -2.253, p=0.044). However, the flight speed of straight flight did not differ between bee species (t87=0.097, p=0.923; Fig. 4).
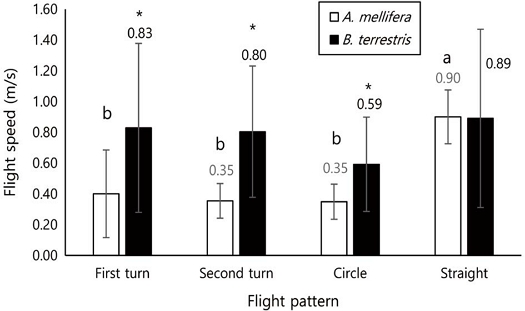
Comparison of Flight speeds between Apis mellifera and Bombus terrestris based in different flight patterns. Error bars are standard deviation values. “*” indicates that the bee species data were significantly different according to a t-test (p<0.05). Different letters indicate significant differences among flight patterns, based on the results of one-way ANOVA and Tukey’s HSD post-hoc test (p<0.05).
By behavior of bees leaving or returning to the hive, the speed of some flight patterns showed a difference (Table 7). In case of honeybees, the one-turn and straight flight patterns in flight leaving the hive were 2.5 times and 1.4 times faster than return flights, respectively (t71= -4.288, p=0.0001; t46= -3.974, p=0.0001; Table 7). In contrast, there was no significant difference between arrival and departure speeds in the case of bumblebees (p>0.05; Table 7).
DISCUSSION
In this study, we investigated the flight speeds and patterns of honeybees and bumblebees near their hives as a basis for making an economical bee traffic measurement machine. We had to set the optimal frame rate (FPS) to effectively recognize the image of a flying bee nearby a beehive. Therefore, we checked whether the flight speed of bees varies by the distance from the beehive.
In general, when honeybees and bumblebees arrive at their flight destination, they reduce their flight speed linearly with distance so that they can land smoothly without being affected by impact stress (Baird et al., 2013; Chang et al., 2016). When returning to the hive, the bees decreased their flight speed as they approached the hive entrance (Table 3). When bees left the hive, they tended to increase their speed as the flight distance increased, which indicates acceleration. We confirmed that the distance from the hive and the flight speed increased linearly (Fig. 3). Baird et al. (2013) and Linander et al. (2017) reported that the size of the optic flow (the speed of image motion on the retina) is kept constant when honeybees and bumblebee reach their flight destination, thereby reducing their speed. Bumblebees have also been reported to reduce their landing speed to near zero when reaching their destination (Chang et al., 2016; Reber et al., 2016). In addition, the reason for the difference in flight speed or pattern between honeybees and bumblebees may be either differences in the optic flow of information received by bees (Linander et al., 2017) or differences in the amount and frequency of contractions of flying muscles (Hedges et al., 2019; Hickey et al., 2022); however, the exact reason is not yet clear.
Near the hive, bees showed different flight patterns. Generally, bees flew in a straight line, or changed the direction of flight. The patterns of directional changes involved changing the direction once at various angles, changing the direction two or more times in a zigzag or S-shape, flying in a spiral, were observed. In particular, there was a high frequency of complex flights, where the bees changed direction more than once when returning to the hive, while the frequency of simple flights, such as straight lines, was high when leaving the hive. When bees return to the hive, the flight pattern of honeybees is more complex than that of bumblebees. In honeybees, the flight changed direction once or twice, but in bumblebees, flight was either straight or included only one change indirection. When a honeybee enters the hive, tits flight pattern is more complex than that of the bumblebee. The more complex the flight pattern, the slower the flight speed. On the other hand, the simple straight-line flight pattern was more than twice as fast as the other flight patterns. In other words, the closer the bees are to the hive, the slower their flight speed and the more complicated their flight patterns. Therefore, the further away from the hive, the faster the bee’s flight speed and the simpler the flight pattern. In general, there is a correlation between body angle and flight speed in flying insects (Esch et al., 1975; Roharseitz and Fry, 2011; Medici and Fry, 2012). During landing, bees control their propulsion and flight speed by adjusting their body pitch angle (Dudley and Ellington, 1990). The reason for the differed flight speed and patterns of each bee species observed in this study when returning to the hive might be a mechanism to reduce their flight speed by changing the pitch angle of the body, to avoid colliding with the hive structure (Crall et al., 2015).
In summary, the flight speeds and patterns of bees returning to or leaving the hive depended in distance from the hive, and there were also differences between the bee species. The closer the bees were to the hive, the slower their flight speeds and the more complicated their flight patterns were. Therefore, this results of flight speeds near hives will provide information for determining the image frame rates at which bees can be captured effectively. Also, the size of the area for recognizing bees may vary depending on the image frame rate. For example, if a bumblebee leaves its hive at a flight speed of 0.87±0.24 (m/s), and the video shoots at 5 FPS, a minimum area of 22.2 cm long is required. If the image acquisition speed is increased to 10 FPS, the minimum length of the recording area can be reduced to 11.1 cm. However, as the image frame rate increases, the capacity required for image storage, or the CPU performance for recognizing bees in the image, must also be higher. A camera lens that collects images also has different focal lengths and angles of view, depending on the type. Therefore, to put this technology to practical use, the image frame rate should be determined by taking the CPU performance, camera angle of view, and focal length into consideration, within the constraints of a limited budget.
Our study is the first to quantitatively measure the flight patterns and speeds of bees around their hives in the field. We found that the flight patterns of bees entering and leaving the hive near the hive were significantly different. We also confirmed that the flight speed changed depending on the distance from the beehive. This result can be a powerful tool for measuring the entry and exit of bees near a hive. In particular, it will be a useful technique to measure the activity of bees using image deep learning (Lee et al., 2020). In addition, this approach could provide basic data for behavioral studies around beehives and for kinetic dynamics research. In this study, we could not clearly distinguish between foraging flights, or flights that were simply reconnaissance or hovering around the beehive, because the study was conducted in a limited camera field of view. Since flight speeds and patterns can vary depending on the learning capacity of bees (Chittka, 2017), future research should closely examine the flight speeds by classifying foraging bees, reconnaissance or learning bees, as well as comparing experienced and young bees. In addition, since wind affects bees’ food intake and flight patterns (Chang et al., 2016; Oh et al., 2022), the influence of weather conditions on bees’ flight speeds and patterns should also be considered.
Acknowledgments
This work was supported by a grant from the National Institute of Agricultural Sciences, Rural Development Administration, Republic of Korea (Project No.: PJ01953012022).
References
-
Abak, K., N. Sari, M. Paksoy, O. Kaftanoglu and H. Yeninar. 1995. Efficiency of bumble bees on the yield and quality of eggplant and tomato grown in unheated glasshouses. Acta Hortic. 412: 268-274.
[https://doi.org/10.17660/ActaHortic.1995.412.30]
-
Alburaki, M., S. Madella and M. Corona. 2021. RFID technology serving honey bee research: A comprehensive description of a 32-antenna system to study honey bee and queen behavior. Appl. Syst. Innov. 4: 88.
[https://doi.org/10.3390/asi4040088]
-
Baird, E. and M. Dacke. 2012. Visual flight control in naturalistic and artificial environments. J. Comp. Physiol. A. 198: 869-876.
[https://doi.org/10.1007/s00359-012-0757-7]
-
Baird, E., N. Boeddeker, M. R. Ibbotson and M. V. Srinivasan. 2013. A universal strategy for visually guided landing. Proc. Natl. Acad. Sci. USA 110: 18686-18691.
[https://doi.org/10.1073/pnas.1314311110]
-
Boenisch, F., B. Rosemann, B. Wild, D. Dormagen, F. Wario and T. Landgraf. 2018. Tracking all members of a honey bee colony over their lifetime using learned models of correspondence. Front. Robot. AI 5: 35.
[https://doi.org/10.3389/frobt.2018.00035]
-
Chang, J. J., J. D. Crall and S.A. Combes. 2016. Wind alters landing dynamics in bumblebees. J. Exp. Biol. 219: 2819-2822.
[https://doi.org/10.1242/jeb.137976]
-
Chittka, L. 2017. Bee cognition. Curr. Biol. 27: R1049-R1053.
[https://doi.org/10.1016/j.cub.2017.08.008]
-
Costa, L., P. Nunes-Silva, J. S. Galaschi-Teixeira, H. Arruda, J. C. Veiga, G. Pessin, P. de Souza and V. L. Imperatriz-Fonseca. 2021. RFID-tagged Amazonian stingless bees confirm that landscape configuration and nest re-establishment time affect homing ability. Insects Soc. 68: 101-108.
[https://doi.org/10.1007/s00040-020-00802-4]
-
Crall, J. D., S. Ravi, A. M. Mountcastle and S. A. Combes. 2015. Bumblebee flight performance in cluttered environments: effects of obstacle orientation, body size and acceleration. J. Exp. Biol. 218: 2728-2737.
[https://doi.org/10.1242/jeb.121293]
-
Crall, J. D., J. J. Chang, R. L. Oppenheimer and S. A. Combes. 2017. Foraging in an unsteady world: bumblebee flight performance in field-realistic turbulence. Interface Focus 7: 20160086.
[https://doi.org/10.1098/rsfs.2016.0086]
-
Dell, A. I., J. A. Bender, K. Branson, I. D. Couzin, G. G. de Polavieja, L. P. J. J. Noldus, A. Pérez-Escudero, P. Perona, A. D. Straw, M. Wikelski and U. Brose. 2014. Automated image-based tracking and its application in ecology. Trends Ecol. Evol. 29: 417-428.
[https://doi.org/10.1016/j.tree.2014.05.004]
-
Dudley, R. and C. P. Ellington. 1990. Mechanics of forward flight in bumblebees: I. Kinematics and morphology. J. Exp. Biol. 148: 19-52.
[https://doi.org/10.1242/jeb.148.1.19]
-
Esch, H., W. Nachtigall and S. N. Kogge. 1975. Correlations between aerodynamic output, electrical activity in the indirect flight muscles and wing positions of bees flying in a servomechanically controlled wind tunnel. J. Comp. Physiol. 100: 147-159.
[https://doi.org/10.1007/BF00613966]
-
Gallai, N., J. M. Salles, J. Settele and B. E. Vaissière. 2009. Economic valuation of the vulnerability of world agriculture confronted with pollinator decline. Ecol. Econ. 68: 810-821.
[https://doi.org/10.1016/j.ecolecon.2008.06.014]
- Gary, N. E. 1992. Activities and behavior of honey bees. pp. 269-372. in The hive and the honey bee, ed. by J. M. Graham). Dadant and Sons, Hamilton, IL.
-
Hedges, C. P., R. T. Wilkinson, J. B. Devaux and J. R. Hickey. 2019. Hymenoptera flight muscle mitochondrial function: Increasing metabolic power increases oxidative stress. Comp. Biochem. Physiol. Part A Mol. Integr. Physiol. 230: 115-121.
[https://doi.org/10.1016/j.cbpa.2019.01.002]
-
Hickey, T., J. Devaux, V. Rajagopal, A. Power and D. Crossman. 2022. Paradoxes of Hymenoptera flight muscles, extreme machines. Biophys. Rev. 14: 403-412.
[https://doi.org/10.1007/s12551-022-00937-7]
-
Klein, A. M., B. E. Vaissière, J. H. Cane, I. S. Dewenter, S. A. Cunningham and C. Fremen. 2007. Importance of pollinators in changing landscapes for world crops. Proc. R. Soc. B: Biological Sciences 274: 303-313.
[https://doi.org/10.1098/rspb.2006.3721]
-
Klein, S., C. Pasquaretta, X. J. He, C. Perry, E. Søvik, J. M. Devaud, A. B. Barron and M. Lihoreau. 2019. Honey bees increase their foraging performance and frequency of pollen trips through experience. Sci. Rep. 9: 6778.
[https://doi.org/10.1038/s41598-019-42677-x]
-
Kulyukin, V. and S. Mukherjee. 2019. On video analysis of omnidirectional bee traffic: counting bee motions with motion detection and image classification. Appl. Sci. 9: 3743.
[https://doi.org/10.3390/app9183743]
-
Lecoeur, J., M. Dacke, D. Floreano and E. Baird. 2019. The role of optic flow pooling in insect flight control in cluttered environments. Sci. Rep. 9: 7707.
[https://doi.org/10.1038/s41598-019-44187-2]
-
Lee, K. Y., S. Choi, J. Lee and H. J. Yoon. 2020. Development of imaging-based honeybee traffic measurement system and its application to crop pollination. J. Apic. 35: 233-243.
[https://doi.org/10.17519/apiculture.2020.11.35.4.233]
-
Linander, N., E. Baird and M. Dacke. 2017. How bumblebees use lateral and ventral optic flow cues for position control in environments of different proximity. J. Comp. Physiol A. 203: 343-351.
[https://doi.org/10.1007/s00359-017-1173-9]
-
Liu, C., J. J. Leonard and J. J. Feddes. 1990. Automated monitoring of flight activity at a beehive entrance using infrared light sensors. J. Apic. Res. 29: 20-27.
[https://doi.org/10.1080/00218839.1990.11101193]
-
Medici, V. and S. N. Fry. 2012. Embodied linearity of speed control in Drosophila melanogaster. J. R. Soc. Interface 9: 3260-3267.
[https://doi.org/10.1098/rsif.2012.0527]
-
Meikle, W. G. and N. Holst. 2015. Application of continuous monitoring of honeybee colonies. Apidologie 46: 10-22.
[https://doi.org/10.1007/s13592-014-0298-x]
-
Oh, D., J. H. Choi, N. H. Kim, D. Kim and K. W. Kim. 2022. Effect of the presence or absence of wind on food intake of eastern honeybees, Apis cerana. J. Apic. 37: 199-206.
[https://doi.org/10.17519/apiculture.2022.09.37.3.199]
-
Ravi, S., O. Bertrand, T. Siesenop, L.-S. Manz, C. Doussot, A. Fisher and M. Egelhaaf. 2019. Gap perception in bumblebees. J. Exp. Biol. 222: jeb184135.
[https://doi.org/10.1242/jeb.184135]
-
Reber, T., M. Dacke, E. Warrant and E. Baird. 2016. Bumblebees perform well-controlled landings in dim light. Front. Behav. Neurosci. 10: 174.
[https://doi.org/10.3389/fnbeh.2016.00174]
-
Rohrseitz, N. and S. N. Fry. 2011. Behavioural system identification of visual flight speed control in Drosophila melanogaster. J. R. Soc. Interface 8: 171-185.
[https://doi.org/10.1098/rsif.2010.0225]
-
Seidl, R., D. Thom, M. Kautz, D. Martin-Benito, M. Peltoniemi, G. Vacchiano, J. Wild, D. Ascoli, M. Petr, J. Honkaniemi, M. J. Lexer, V. Trotsiuk, P. Mairota, M. Svoboda, M. Fabrika, T. A. Nagel and C. P. O. Reyer. 2017. Forest disturbances under climate change. Nat. Clim. Change 7: 395-402.
[https://doi.org/10.1038/nclimate3303]
-
Struye., M. H., H. J. Mortier, G. Arnold, C. Miniggio and R. Borneck. 1994. Microprocessor-controlled monitoring of honeybee flight activity at the hive entrance. Apidologie 25: 384-395.
[https://doi.org/10.1051/apido:19940405]
-
Sun, C. and P. Gaydeck. 2021. A visual tracking system for honey bee (Hymenoptera: Apidae) 3D flight trajectory reconstruction and analysis. J. Insect Sci. 21: 17.
[https://doi.org/10.1093/jisesa/ieab023]
-
Velthuis, H. H. W. and A. van Doorn. 2006. A century of advances in bumblebee domestication and the economic and environmental aspects of its commercialization for pollination. Apidologie 37: 421-451.
[https://doi.org/10.1051/apido:2006019]
-
Woodgate, J. L., J. C. Makinson, K. S. Lim, A. M. Reynolds and L. Chittka. 2016. Life-long radar tracking of bumblebees. PLoS One 11: e0160333,
[https://doi.org/10.1371/journal.pone.0160333]
- Yoon, H. J., K. Y. Lee, I. G. Park and M. A. Kim. 2010. Temperature and humidity favorable for colony development of Korean native bumblebee Bombus hypocrita sapporoensis. J. Apic. 25: 83-89.