
플루피라디퓨론 장기노출이 꿀벌 생리에 미치는 영향
Abstract
The Western honey bees, Apis mellifera, play an essential role in pollinating both commercial crops and wild plants. While foraging, honey bees are exposed to diverse kinds of pesticides via oral ingestion or contact. Several neonicotinoids such as imidacloprid, chlothianidin and thiametoxam have posed a severe threat to honey bees with their high chronic toxicity especially when used for seed treatments. Flupyradifurone (FPF), a butanolide insecticide targeting nicotinic acetylcholine receptors of pests, is considered as a good alternative to neonicotinoids as it selectively targets sucking pests without much harming honey bees. In this study, we investigated any negative effects of chronic oral ingestion of FPF on honey bees under an extreme exposure condition. Foragers chronically exposed to 10 ppm of FPF showed difference in neither the flight ability nor the weight of thoraces. However, transcriptome analysis of foragers revealed increased protein translation/modification and lipid metabolism in the thorax and abdomen, respectively. These results, along with the increase in whole bodyweight, suggest that chronic exposure to high doses of FPF can induce obesity and oxidative stress in honey bees. Nevertheless, our finding does not necessarily mean that field-realistic doses of FTP under the actual chronic exposure scenario likely cause adverse impacts on honey bees.
Keywords:
Honey bee, Flupyradifurone, Flight ability, Body weight, Protein metabolism, Lipid metabolism서 론
양봉꿀벌 (Apis mellifera, 이하 꿀벌)은 상업작물과 야생식물의 화분매개에 중요한 역할을 하며 (Morse and Calderone, 2000) 그 가치는 미국에서만 2015년 기준 연간 최대 547억 달러에 달한다고 추산된다 (Chisel, 2015). 꿀벌 성충은 우화 후 약 21일부터 활발히 외역활동을 하는데 (Abou-Shaara, 2014) 물, 화분, 화밀의 채집 과정 중 다양한 살충제에 노출된다. 특히 네오니코티노이드계 (neonicotinoid) 살충제와 같은 침투이행성 약제는 종자처리 과정 또는 오염된 대지에서 식물의 수분흡수 과정을 통해 식물 체내에 유입되어 화분 및 화밀로 이동하고, 이를 꿀벌이 운반, 가공 및 섭식하는 과정에서 만성노출이 이루어진다 (Krupke et al., 2012). 네오니코티노이드계 살충제 중에서 특히 이미다클로프리드 (imidacloprid, IMI)는 흡즙성 및 저작해충에 대해 높은 방제효율을 지니고 있어 종자처리에 널리 사용되어 왔다 (Kuhar et al., 2002; Zhang et al., 2011). 그러나 해독작용과 관련된 꿀벌 cytochrome P450의 기질 특이성으로 인해 IMI는 다른 네오니코티노이드계 살충제에 비해 높은 꿀벌 독성을 보인다 (Manjon et al., 2018). IMI는 극미량에서도 꿀벌의 외역활동 (Colin et al., 2019), 면역 (Christen and Fent, 2017), 에너지대사 (Gooley and Gooley, 2020), 발달 (Tome et al., 2020) 등 생리 전반에 영향을 주는 것으로 알려져 있어 전 세계적으로 사용이 감소되고 있는 추세이다.
플루피라디퓨론 (flupyradifurone, FPF)은 종합적해충관리 (integrated pest management, IPM)를 위해 독일 Bayer사에서 개발한 살충제로, 2014년 Sivanto라는 제품명으로 출시되었다. 뷰테놀라이드계 (Butenolide) 살충제로서 네오니코티노이드계 살충제와 마찬가지로 곤충의 니코틴성 아세틸콜린 수용체에 작용하나, 구조가 상이하여 IMI 대사에 관여하는 CYP6CM1에 의해 분해되지 않는 특성이 있다 (Jeschke et al., 2015; Nauen et al., 2015). FPF 역시 침투이행성 약제로 흡즙성 해충에 높은 방제 효율을 보이나, 꿀벌에 대한 선택독성은 극히 낮아서 급성 섭식 반수치사량 (LD50)이 IMI의 300배 이상에 이르는 저독성을 보인다 (Haas et al., 2021). 따라서 FPF는 종자처리뿐만 아니라 개화시기에 엽면 살포 또한 가능한데 (Nauen et al., 2015), 개화시기에 권장량의 FPF가 엽면 살포된 메밀밭 인근의 꿀벌 봉군은 대조군의 꿀벌 봉군 세력 지표에서 차이를 보이지 않았으며 (Campbell et al., 2016), 포장 검출 농도에서 단기 및 장기 노출에도 꿀벌의 설탕 섭식반응에 변화가 없었다 (Bell et al., 2020a).
그러나 꿀벌에 부정적 영향을 미친다는 연구결과도 보고되고 있다. 포장 검출 농도 (~4 ppm)의 FPF에 노출된 꿀벌들의 생존율, 외역활동, 학습 및 기억력이 감소됐으며 후각, 해독 관련 유전자 및 인슐린 수용체의 발현 변화가 보고되었다 (Bell et al., 2020b; Guo et al., 2021; Tosi et al., 2021; Wu et al., 2021). 포장 처리 농도에서는 꿀벌의 산화스트레스를 증가시키고 apoptosis를 유발시키는 것으로 알려져 있다 (Chakrabarti et al., 2020). 첫 시판으로부터 10년이 채 되지 않아 FPF 노출이 꿀벌 봉군에 미치는 영향에 대한 다각적 접근이 필요한 시점이다.
본 연구에서는 FPF가 꿀벌에 만성노출이 될 극한적인 경우를 가정하고 꿀벌에 미치는 영향을 조사하고자 하였다. 처리농도는 FPF가 엽면 살포된 유채 포장에서 외역을 수행하는 일벌이 수집한 화분에서 검출된 농도인 21 ppm의 절반인 10 ppm으로 설정하여 설탕물 및 화분떡에 섞어 장기 급여하였다 (Carleton, 2014). 꿀벌의 외역활동에 미치는 영향을 파악하기 위해 Flight mill을 통해 비행능력을 측정하였고 생리변화의 지표로 체중 측정 및 전사체 분석을 통한 대사경로 분석을 진행하였다.
재료 및 방법
1. FPF 처리 및 꿀벌 시료 채집
본 실험은 2019년 5월 하순에 시작하였다. 실험에 사용된 양봉꿀벌 (Apis mellifera)은 모계가 동일한 이탈리안 잡종으로 여섯 개의 봉군을 양봉업자로부터 구매하여 서울대학교 관악캠퍼스 농업생명과학대학 옥상 (Seoul, Korea, 37°27ʹ46.8ʺN, 126°57ʹ06.9ʺE)에 위치한 양봉장에 비치하였다. 여섯 봉군은 모두 비슷한 군세로 6매 벌집 (소비)에 약 15,000마리의 일벌로 이루어졌다. 처리구는 FPF 처리군과 무처리 대조군으로 세 반복으로 수행되었다. 분석표준 순도의 FPF는 Merck사 (Darmstadt, Germany)에서 구매하여 아세톤에 10,000 ppm 농도로 용해시켜 저장 용액을 만들었다. 해당 용액을 대용화분 (불로양봉원, 남양주, 대한민국) 및 당액 (설탕 : 물=5 : 7)에 1 : 1000 비율로 혼합하여 최종 10 ppm의 농도로 FPF 처리군에 공급하였으며 100% 아세톤을 동일 비율로 혼합한 당액 및 대용화분을 대조군에 공급하였다. 대용화분은 0일차부터 일주일 간격으로 400 g씩 공급하였으며 80% 이상 섭식했을 시 바로 보충하였다. 당액은 실험 0, 10, 20, 30, 40일차에 각 봉군에 1 kg씩 총 5회 공급하였다.
일벌 연령을 통일하기 위해 벌집이 지어진 빈 벌집 2매를 넣은 여왕 격리통에 각 여왕을 이틀간 가두어 산란을 받았다. 23일 후, 봉개된 소비들을 인큐베이터에 (34°C, 상대습도 80%) 보관하여 우화한 일벌을 확보하였다. 갓 우화한 일벌들은 봉군별 1000마리 이상 가슴 등쪽에 마커 (Uni posca, Mitsubishi Pencil, Japan)로 대조군, 실험군별로 다르게 표지하여 본 봉군으로 풀어주었으며 일부는 체중을 측정하였다 (반복당 10마리, 봉군당 3반복으로 총 9반복). 실험 32일차 및 46일차에 7일령 일벌과 21일령 일벌을 포획하여 체중 측정 후 액체질소로 급속 냉동하여 -80°C에 보관하였다. 비행능력 비교를 위해서 26~32일령 일벌을 포획하여 flight mill 실험을 수행하였다.
또한, 꿀벌 비행근육 발달 비교를 위해 21일령 대조군 16마리, FPF 처리군 14마리의 가슴을 분리하여 개별 무게를 측정하였다.
2. 비행 능력 조사
비행 능력 조사를 위해서 flight mill을 제작하여 사용하였다 (Kim et al., 2022b). 두 자석의 같은 극을 붙여 부상시켜 마찰을 줄인 회전기구에 꿀벌을 부착하여 한 바퀴 회전 시 걸리는 시간을 아두이노 (Arduino) 시스템에 연결된 자기 센서를 통해 측정 및 기록하였다. 포획한 일벌은 더 이상 날지 않을 때까지 flight mill에서 탈진시키고 10분의 휴식 뒤 1 M 포도당 수용액 10 μL를 먹인 후 비행거리, 비행시간 및 평균속도를 측정하였다. 연속 비행시간이 2분 미만이거나 1시간 이상이거나 비행거리가 200 m 미만인 경우 결과에서 배제하였다. 비행 능력 조사에 사용된 꿀벌은 대조군이 124마리, FPF 처리군이 118마리였다.
3. 전사체 분석
대조군 및 FPF 처리군 각각 8마리의 21일령 일벌 가슴과 장이 제거된 복부 시료를 취합한 후 TRI reagent (MRC, OH, USA)를 이용하여 total RNA를 추출하였다. Bioanalyzer 2100 system (Agilent Technologies, CA, USA)의 RNA Nano 6000 Assay Kit로 RNA의 순도와 정량을 측정하여 총 1 μg의 RNA를 전사체 분석에 사용하였다. NEBNext® UltraTM RNA. A Library Prep Kit for Illumina® (New England Biolabs, MA, USA)를 이용하여 시퀀싱 라이브러리를 제작하고 index code를 각 시료에 붙여 분석에 사용하였다. 준비된 라이브러리는 Illumina Novaseq6000기기로 시퀀싱하여 2×150 bp의 paired-end reads를 확보하였고 Q<20의 read는 제거하였다. Trimmomatic을 이용하여 trimming 및 adapter를 제거하였다 (Bolger et al., 2014). Trimming된 reads는 Bowtie2 ver 2.3.5를 이용하여 기본 설정값으로 꿀벌 게놈 서열인 Amel_HAv3.1에 정렬하였다 (Langmead and Salzberg, 2012). FeatureCounts ver. 1.6.4로 각 유전자에 맵핑된 read의 수를 정량하였고 (Liao et al., 2014), DESeq2 ver. 1.12.0을 이용하여 대조군과 FPF 처리군 간의 차등발현 유전자 (Differentially expressed gene, DEG)의 발현량을 정규화하였다 (Love et al., 2014). 발현량 차이는 1.5배 (|Log2FC|≥0.6, p<0.05)를 기준으로 DEG를 선정하여 가슴과 복부에서 각각 상향조절 (upregulation)되거나 하향조절 (downregulation)된 DEG 리스트를 g:Profiler의 g:GOSt (https://biit.cs.ut.ee/gprofiler/gost)에 업로드하여 유의미하게 변화된 Gene ontology (GO) 및 Kyoto Encyclopedia of Genes and Genomes (KEGG) 대사경로 (padj<0.05)를 확보하였다 (Raudvere et al., 2019).
4. 통계 분석
대조군과 FPF 처리군 간의 통계적 차이를 보기 위해 스튜던트 t-검정을 사용하였다. 일령 간 일벌의 체중 비교를 위해서는 일원분산분석 (one-way ANOVA)를 이용하고 Tukey 사후검정으로 나이별 체중 간 유의성을 검증하였다. 모든 통계처리와 그래프 제작은 Prism 6.0 (GraphPad, San Diego, USA)을 이용하였다.
결과 및 고찰
1. FPF 처리에 따른 꿀벌 체중 변화
갓 우화한 일벌, 7일령 일벌 (내역봉), 21일령 일벌 (외역봉)의 체중을 측정하였다 (Fig. 1). 각 실험군 내 연령별로 비교한 경우, 대조군과 FPF 처리군 모두 갓 우화한 일벌에서 내역봉으로 성장 시에는 체중이 증가하나 (p<0.0001, p=0.0004), 내역봉에서 외역봉으로 성장 시에는 감소하여 (p<0.0001, p=0.0006) 갓 우화한 일벌과 외역봉 간에는 유의미한 차이가 없었다 (p=0.15, p=0.98). 대조군과 FPF 처리군 간 체중을 비교한 결과, 갓 태어난 일벌 (p=0.67)과 내역봉 (p=0.54)에서는 유의미한 차이를 보이지 않았지만 외역봉에서는 평균 체중이 각각 101.5±1.8 및 111.0±3.2 mg으로 FPF 처리군에서 유의미하게 체중이 증가하였다 (p=0.02). 이와 같은 체중 증가 경향은 IMI 100 ppb에 장기노출된 외역봉에서도 보고된 바 있다 (Kim et al., 2022b).
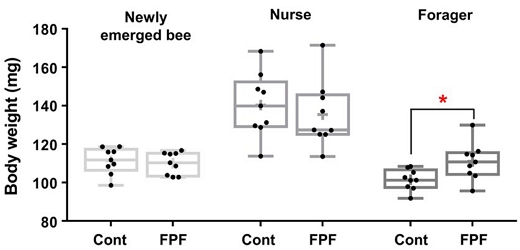
Body weights of the honey bees. Nine replications of 10-pooled honey bees were weighed for each condition, and the weights were divided by 10 to calculate the weight of single honey bee. The line in the box and+were plotted at the median and the average, respectively. Significant differences are marked with * (p<0.05).
2. FPF 처리에 따른 꿀벌 비행능력 변화
꿀벌 비행능력 검정에 사용된 대조군 124마리, FPF 처리군 118마리의 외역봉에서 각각 17마리, 12마리의 비행 데이터를 확보하였다. 확보된 데이터로부터 비행거리, 비행시간 및 평균속도를 산출하였다 (Fig. 2). 대조군, FPF 처리군별 총 비행거리는 1074±62 m 및 1078±94 m (p=0.97), 총 비행시간은 959±45초 및 877±71초 (p=0.32), 평균속도는 1.12±0.06 m/s 및 1.24±0.09 m/s (p=0.26)로 모두 유의미한 차이가 없어 10 ppm 농도의 FPF 노출은 꿀벌의 비행능력에 큰 영향을 미치지 않는 것으로 사료된다. 본 실험의 10 ppm FPF 처리와 마찬가지로 외역봉의 체중을 증가시킨 100 ppb IMI 노출에는 꿀벌의 비행능력이 감소되었던 반면 (Kim et al., 2022b), 4 ppm 농도의 FPF에 노출된 꿀벌은 외역활동에 차이가 없는 것으로 보고된 바 있다 (Tong et al., 2019; Guo et al., 2021). 그러나 FPF는 계절, 꿀벌의 나이, 영양상태에 따라 꿀벌의 운동성, 비행능력, 행동 등에 영향을 미치는 것으로 알려져 있어 FPF에 의한 영향평가 시 환경변수에 대한 고려가 요구된다 (Hesselbach and Scheiner, 2019; Tong et al., 2019; Tosi and Nieh, 2019).
3. FPF 처리에 따른 꿀벌 대사경로 변화
1.5배 발현차이를 기준으로 DEG를 분석한 결과, FPF 처리군에서 가슴에서는 162개 및 68개의 유전자 발현이 각각 상향조절 및 하향조절되었으며 복부에서는 62개 및 92개의 유전자 발현이 각각 상향조절 및 하향조절됨을 확인하였다 (Table 1).
해당 DEG 리스트로 functional annotation을 수행한 결과, 가슴에서는 40개 및 1개의 대사경로가 상향조절 및 하향조절되었으며 복부에서는 6개 및 1개의 대사경로가 상향조절 및 하향조절되었다 (Table S1). 가슴에서 상향조절된 주요대사경로는 biological process (BP)에서 ‘Translation (GO:0006212)’, cellular component (CC) 및 KEGG에서 ‘Ribosome (GO:0005840, KEGG:03010)’, molecular function (MF)에서 ‘Structural constituent of ribosome (GO:0003735)’ 등 대부분 단백질 translation 및 modification에 관련돼 있었다 (Fig. 3A). 외역봉에서 가슴을 따로 분리하여 무게를 측정한 결과 대조군과 FPF 처리군 간 가슴 무게의 차이는 없어 단백질 대사경로의 증가가 실제 근육 증가로 이어지지는 않은 것으로 추정된다 (Fig. 4). 살충제 노출은 세포 내부의 산화스트레스를 증가시키고 이는 정상적인 단백질 translation 및 modification을 저해한다 (Abdollahi et al., 2004; Shenton et al., 2006; Gregersen and Bross, 2010). 꿀벌의 경우, IMI 노출 농도가 증가할수록 단백질 관련 대사가 증가하였으며 IMI 20 ppb 노출 시에도 마찬가지로 산화스트레스를 유발하는 고온조건과 함께 처리될 경우 단백질 관련 대사의 증가가 보고된 바 있다 (Kim et al., 2022a, b). 이는 10 ppm 농도의 FPF 노출이 비행능력에 영향을 주지는 않았지만 꿀벌에 산화스트레스를 주고 있다는 것을 시사한다. 가슴에서 하향조절된 대사경로는 ‘Tryptophan metabolism (KEGG:00380)’ 1개로 (Fig. 3B) tryptophan 대사경로는 살충제 반응에 중요한 역할을 하는 것으로 알려져 있어 FPF가 다른 살충제와 시너지 효과를 일으킬 가능성을 제시한다 (Brinzer et al., 2015).

Functional annotation of the transcriptome data. DEGs between the FPF-treated group versus the control group with the criteria of |Log2FC|≥0.6 and p<0.05 were used as input data. Top three pathways based on padj values from each classification (biological process, BP; cellular component, CC; molecular function, MF; KEGG) were represented.
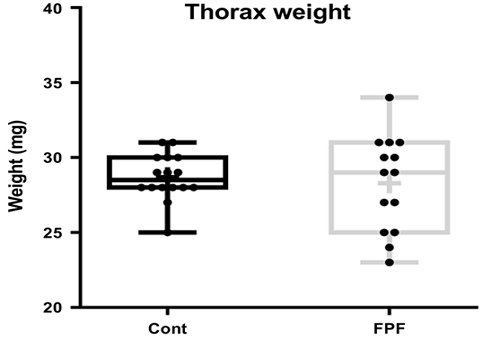
Weight of forager thoraces. 16 and 14 units from the control group and the FPF-treated group were weight. The line in the box and+were plotted at the median and the average, respectively.
복부에서는 BP에서 ‘Lipid metabolic process (GO: 0006629)’, KEGG에서 ‘Valine, leucine and isoleucine biosynthesis (KEGG:00290)’, MF에서 ‘Fatty acid elongase activity (GO:0009922)’ 등의 대사경로가 FPF 처리에 상향조절되었다 (Fig. 3C). 지질관련 대사의 증가는 외역봉의 체중 증가 (Fig. 1)와 밀접한 관련이 있는 것으로 추정된다. 기존 연구에서 IMI 100 ppb에 노출된 외역봉 또한 체중 증가에 따른 복부에서의 지질대사 증가 및 지질 비율 증가를 보였는데 인슐린 저항성이 그 원인으로 지목되었다 (Kim et al., 2022b). 네오니코티노이드계와 피레스로이드계 (pyrethroid)와 같은 살충제는 세포의 탈분극을 일으켜 triglyceride의 축적을 유도하여 비만 및 제2형 당뇨를 유발하는 것으로 추정되는데 (Park et al., 2022), 이들 살충제와 유사하게 탈분극을 일으키는 FPF 또한 10 ppm의 높은 농도에서는 비만 및 인슐린 저항성을 유도하는 것으로 추정된다. 종합하자면 꿀벌이 10 ppm FPF에 장기적으로 노출될 경우 악영향을 받을 수 있을 것으로 예상된다. 그러나 본 연구에서 얻어진 결과는 비교적 높은 농도의 FPF를 장기간 만성적으로 노출시키는 극한적 가정을 전제한 것이므로, 실제의 만성노출 시나리오에서 예상되는 극미량의 FPF가 꿀벌에 미치는 부정적 영향은 제한적일 것으로 사료된다. FPF의 만성노출에 따른 보다 정확한 위해성 평가를 위해서는 종자처리나 엽면살포 시 화밀과 화분으로 이행하는 FPF의 잔류량을 조사한 후 이들 약량 수준에 만성노출된 꿀벌의 생리적 변화에 대한 연구가 필요하다 하겠다.
적 요
본 연구에서는 종자처리나 엽면살포에 의해 화분 및 꿀에 유입된 FPF의 꿀벌에 대한 독성평가를 수행하고자 하였다. 이를 위해 대조군 및 FPF 처리군으로 각각 3통의 봉군을 야외포장에 두고 FPF를 10 ppm 농도로 화분떡 및 설탕물에 섞어 봉군에 장기 급여하였다. 그 결과, 갓 태어난 일벌 및 내역봉에서는 체중 변화가 없었지만 외역봉에서는 체중이 증가하는 추세를 보였다. 외역봉의 전사체 분석 결과 FPF 섭식으로 인해 가슴에서는 단백질 translation 및 modification 관련 대사경로가 상향조절되었으며 복부에서는 지질대사경로가 하향조절되었는데, 이는 각각 산화스트레스와 인슐린 저항성 발달/비만과 관련된 것으로 예상된다. 외역봉의 비행능력은 FPF 처리에 의해 영향을 받지 않았지만 체중 및 전사체 분석 결과로 미루어 볼 때 10 ppm FPF 장기노출은 꿀벌 생리를 악화시키는 것으로 보인다. 그러나 본 연구 결과는 꿀벌이 비교적 높은 농도 (10 ppm)의 FPF에 장기간 노출되는 극한적 가정을 전제한 것이므로, 실제의 만성노출 시나리오에서 예상되는 극미량의 FPF가 꿀벌에 미치는 부정적 영향은 제한적일 것으로 사료된다.
Acknowledgments
본 연구는 농촌진흥청 (Rural Development Administration) 연구사업 (PJ015763)의 지원을 받아 수행되었다.
References
- Abdollahi, M., A. Ranjbar, S. Shadnia, S. Nikfar and A. Rezaie. 2004. Pesticides and oxidative stress: a review. Med. Sci. Monit. 10: 141-147.
-
Abou-Shaara, H. F. 2014. The foraging behaviour of honey bees, Apis mellifera: a review. Vet. Med. 59.
[https://doi.org/10.17221/7240-VETMED]
-
Bell, H. C., J. E. Benavides, C. N. Montgomery, J. R. E. Navratil and J. C. Nieh. 2020a. The novel butenolide pesticide flupyradifurone does not alter responsiveness to sucrose at either acute or chronic short-term field-realistic doses in the honey bee, Apis mellifera. Pest. Manag. Sci. 76: 111-117.
[https://doi.org/10.1002/ps.5554]
-
Bell, H. C., C. N. Montgomery, J. E. Benavides and J. C. Nieh. 2020b. Effects of Nosema ceranae (Dissociodihaplophasida: Nosematidae) and flupyradifurone on olfactory learning in honey bees, Apis mellifera (Hymenoptera: Apidae). J. Insect. Sci. 20: 29.
[https://doi.org/10.1093/jisesa/ieaa130]
-
Bolger, A. M., M. Lohse and B. Usadel. 2014. Trimmomatic: a flexible trimmer for Illumina sequence data. Bioinformatics 30: 2114-2120.
[https://doi.org/10.1093/bioinformatics/btu170]
-
Brinzer, R. A., L. Henderson, A. A. Marchiondo, D. J. Woods, S. A. Davies and J. A. Dow. 2015. Metabolomic profiling of permethrin-treated Drosophila melanogaster identifies a role for tryptophan catabolism in insecticide survival. Insect Biochem. Mol. Biol. 67: 74-86.
[https://doi.org/10.1016/j.ibmb.2015.09.009]
-
Campbell, J. W., A. R. Cabrera, C. Stanley-Stahr and J. D. Ellis. 2016. An evaluation of the honey bee (Hymenoptera: Apidae) safety profile of a new systemic insecticide, flupyradifurone, under field conditions in Florida. J. Econ. Entomol. 109: 1967-1972.
[https://doi.org/10.1093/jee/tow186]
- Carleton, J. 2014. Environmental fate and ecological risk assessment for foliar, soil drench, and seed treatment uses of the new insecticide flupyradifurone (BYI 029 60). Licensee MDPI, Basel, Switzerland. This article is an open access article distributed under the terms and conditions of the Creative Commons Attribution (CC BY) license (http://creativecommons.org/licenses/by/4.0/, ).
-
Chakrabarti, P., E. A. Carlson, H. M. Lucas, A. P. Melathopoulos and R. R. Sagili. 2020. Field rates of SivantoTM (flupyradifurone) and Transform® (sulfoxaflor) increase oxidative stress and induce apoptosis in honey bees (Apis mellifera L.). PLos One 15: e0233033.
[https://doi.org/10.1371/journal.pone.0233033]
- Chisel, J. T. 2015. Honey Bees’ Impact on the US Economy.
-
Christen, V. and K. Fent. 2017. Exposure of honey bees (Apis mellifera) to different classes of insecticides exhibit distinct molecular effect patterns at concentrations that mimic environmental contamination. Environ. Pollut. 226: 48-59.
[https://doi.org/10.1016/j.envpol.2017.04.003]
-
Colin, T. O., W. G. Meikle, X. Wu and A. B. Barron. 2019. Traces of a neonicotinoid induce precocious foraging and reduce foraging performance in honey bees. Environ. Sci. Technol. 53: 8252-8261.
[https://doi.org/10.1021/acs.est.9b02452]
- Gooley, Z. C. and A. C. Gooley. 2020. Exposure to field realistic concentrations of imidacloprid at different ambient temperatures disrupts non-flight metabolic rate in honey bee (Apis mellifera) foragers. Bull. Insectol. 73: 161-170.
-
Gregersen, N. and P. Bross. 2010. Protein misfolding and cellular stress: an overview. Protein misfolding and cellular stress in disease and aging, 3-23.
[https://doi.org/10.1007/978-1-60761-756-3_1]
-
Guo, Y., Q.-Y. Diao, P.-L. Dai, Q. Wang, C.-S. Hou, Y.-J. Liu, L. Zhang, Q.-H. Luo, Y.-Y. Wu and J. Gao. 2021. The effects of exposure to flupyradifurone on survival, development, and foraging activity of honey bees (Apis mellifera L.) under field conditions. Insects 12: 357.
[https://doi.org/10.3390/insects12040357]
-
Haas, J., M. Zaworra, J. Glaubitz, G. Hertlein, M. Kohler, A. Lagojda, B. Lueke, C. Maus, M.-T. Almanza and T. E. Davies. 2021. A toxicogenomics approach reveals characteristics supporting the honey bee (Apis mellifera L.) safety profile of the butenolide insecticide flupyradifurone. Ecotoxicol. Environ. Saf. 217: 112247.
[https://doi.org/10.1016/j.ecoenv.2021.112247]
-
Hesselbach, H. and R. Scheiner. 2019. The novel pesticide flupyradifurone (Sivanto) affects honeybee motor abilities. Ecotoxicology 28: 354-366.
[https://doi.org/10.1007/s10646-019-02028-y]
-
Jeschke, P., R. Nauen, O. Gutbrod, M. E. Beck, S. Matthiesen, M. Haas and R. Velten. 2015. Flupyradifurone (SivantoTM) and its novel butenolide pharmacophore: Structural considerations☆. Pestic. Biochem. Physiol. 121: 31-38.
[https://doi.org/10.1016/j.pestbp.2014.10.011]
-
Kim, S., S. Cho and S. H. Lee. 2022a. Synergistic effects of imidacloprid and high temperature on honey bee colonies. Apidologie 53: 1-17.
[https://doi.org/10.1007/s13592-022-00980-z]
-
Kim, S., J. H. Kim, S. Cho, J. M. Clark and S. H. Lee. 2022b. Chronic exposure to field-realistic doses of imidacloprid resulted in biphasic negative effects on honey bee physiology. Insect Biochem. Mol. Biol. 144: 103759.
[https://doi.org/10.1016/j.ibmb.2022.103759]
-
Krupke, C. H., G. J. Hunt, B. D. Eitzer, G. Andino and K. Given. 2012. Multiple routes of pesticide exposure for honey bees living near agricultural fields. PLoS One 7: e29268.
[https://doi.org/10.1371/journal.pone.0029268]
-
Kuhar, T. P., L. J. Stivers-Young, M. P. Hoffmann and A. G. Taylor. 2002. Control of corn flea beetle and Stewart̓s wilt in sweet corn with imidacloprid and thiamethoxam seed treatments. Crop. Prot. 21: 25-31.
[https://doi.org/10.1016/S0261-2194(01)00056-4]
-
Langmead, B. and S. L. Salzberg. 2012. Fast gapped-read alignment with Bowtie 2. Nat. Methods 9: 357-359.
[https://doi.org/10.1038/nmeth.1923]
-
Liao, Y., G. K. Smyth and W. Shi. 2014. featureCounts: an efficient general purpose program for assigning sequence reads to genomic features. Bioinformatics 30: 923-930.
[https://doi.org/10.1093/bioinformatics/btt656]
-
Love, M. I., W. Huber and S. Anders. 2014. Moderated estimation of fold change and dispersion for RNA-seq data with DESeq2. Genome Biol. 15: 1-21.
[https://doi.org/10.1186/s13059-014-0550-8]
-
Manjon, C., B. J. Troczka, M. Zaworra, K. Beadle, E. Randall, G. Hertlein, K. S. Singh, C. T. Zimmer, R. A. Homem and B. Lueke. 2018. Unravelling the molecular determinants of bee sensitivity to neonicotinoid insecticides. Curr. Biol. 28: 1137-1143. e1135.
[https://doi.org/10.1016/j.cub.2018.02.045]
- Morse, R. A. and N. W. Calderone. 2000. The value of honey bees as pollinators of US crops in 2000. Bee Culture 128: 1-15.
-
Nauen, R., P. Jeschke, R. Velten, M. E. Beck, U. Ebbinghaus-Kintscher, W. Thielert, K. Wölfel, M. Haas, K. Kunz and G. Raupach. 2015. Flupyradifurone: a brief profile of a new butenolide insecticide. Pest Manage. Sci. 71: 850-862.
[https://doi.org/10.1002/ps.3932]
-
Park, Y., A. Suvorov, S. B. Symington and J. M. Clark. 2022. Membrane polarization in non-neuronal cells as a potential mechanism of metabolic disruption by depolarizing insecticides. Food Chem. Toxicol. 112804.
[https://doi.org/10.1016/j.fct.2021.112804]
-
Raudvere, U., L. Kolberg, I. Kuzmin, T. Arak, P. Adler, H. Peterson and J. Vilo. 2019. g: Profiler: a web server for functional enrichment analysis and conversions of gene lists (2019 update). Nucleic. Acids Res. 47: W191-W198.
[https://doi.org/10.1093/nar/gkz369]
-
Shenton, D., J. B. Smirnova, J. N. Selley, K. Carroll, S. J. Hubbard, G. D. Pavitt, M. P. Ashe and C. M. Grant. 2006. Global translational responses to oxidative stress impact upon multiple levels of protein synthesis. J. Biol. Chem. 281: 29011-29021.
[https://doi.org/10.1074/jbc.M601545200]
-
Tome, H. V., D. R. Schmehl, A. E. Wedde, R. S. Godoy, S. V. Ravaiano, R. N. Guedes, G. F. Martins and J. D. Ellis. 2020. Frequently encountered pesticides can cause multiple disorders in developing worker honey bees. Environ. Pollut. 256: 113420.
[https://doi.org/10.1016/j.envpol.2019.113420]
-
Tong, L., J. C. Nieh and S. Tosi. 2019. Combined nutritional stress and a new systemic pesticide (flupyradifurone, Sivanto®) reduce bee survival, food consumption, flight success, and thermoregulation. Chemosphere 237: 124408.
[https://doi.org/10.1016/j.chemosphere.2019.124408]
-
Tosi, S. and J. Nieh. 2019. Lethal and sublethal synergistic effects of a new systemic pesticide, flupyradifurone (Sivanto®), on honeybees. Proc. Royal Soc. B 286: 20190433.
[https://doi.org/10.1098/rspb.2019.0433]
-
Tosi, S., J. C. Nieh, A. Brandt, M. Colli, J. Fourrier, H. Giffard, J. Hernández-López, V. Malagnini, G. R. Williams and N. Simon-Delso. 2021. Long-term field-realistic exposure to a next-generation pesticide, flupyradifurone, impairs honey bee behaviour and survival. Commun. Biol. 4: 1-9.
[https://doi.org/10.1038/s42003-021-02336-2]
-
Wu, Y.-Y., P. Pasberg, Q.-Y. Diao and J. C. Nieh. 2021. Flupyradifurone reduces nectar consumption and foraging but does not alter honey bee recruitment dancing. Ecotoxicol. Environ. Saf. 207: 111268.
[https://doi.org/10.1016/j.ecoenv.2020.111268]
-
Zhang, L., S. M. Greenberg, Y. Zhang and T. X. Liu. 2011. Effectiveness of thiamethoxam and imidacloprid seed treatments against Bemisia tabaci (Hemiptera: Aleyrodidae) on cotton. Pest Manag. Sci. 67: 226-232.
[https://doi.org/10.1002/ps.2056]