
벌집 적용을 위한 폴리프로필렌/밀납 복합재의 FDM 방식 3D 프린팅 연구
Abstract
Polypropylene (PP) polymer compounds were formulated to fabricate reusable beehives with inexpensive material using 3D printing technology. To improve honeybee affinity to plastic comb foundation, bee-friendly materials must be mixed with PP polymer, which correspond to beeswax, talc, woodflour and compatibilizer. The compounds were extruded with 1.75 mm thickness for 3D filaments. The composite materials were observed through SEM to assess the morphology of additives and fillers. The mechanical and thermal properties of the composite were measured using UTM and DSC, respectively and their crystallinity was evaluated by XRD. The crystallinity of the PP polymer was reduced by the addition of beeswax. It was found that the 3D printing compatibility of PP compound increased due to the lowered crystallinity by adding beeswax. Therefore, the optimization of printing condition has to be considered for PP’s shrinkage behavior due to its crystallinity. The PP compounds were printed with 3 different compositions to evaluate dimensional accuracy, deformation rate and warp-index. The shrinkage of PP compound was significantly reduced with the addition of beeswax and compatibilizer. The printing variables were set as nozzle temperature, bed temperature and printing speed. The manufactured honeycomb foundation will be field-tested to examine whether bees can build a nest with good affinity.
Keywords:
Beeswax, Polypropylene, Composite material, Additive manufacturing, Warpage서 론
양봉에서 사용되는 인공소초로서 소비 형성 시 벌 친화성이 높은 밀납 (beeswax)은 천연 고분자 재료로 밀도 0.958~0.970 kg/m3와 융점 62~64℃의 비결정성 물질이며, 화학구조는 C15H31COOC30H61로 palmitate, palmitoleate 및 oleate ester가 포함된 탄소갯수가 30~32개의 긴 사슬로 구성된 지방족 알코올 구조를 가지고 있다 (Abdikheibari et al., 2015; Fratini et al., 2016). 밀납은 소수성의 천연 방수물질로 높은 온도의 알코올 및 클로로폼에서 융해되는 특성을 가지고 살균 효과가 있어 오랫동안 의약 및 미용 목적으로도 사용되어 왔다. 밀납 재질의 소초는 사출 및 롤러 방식을 이용하여 허니콤 형태로 제작되는데, 이러한 소초는 소비 형성 후 채밀 과정에서 형태가 붕괴되어 재활용이 불가능하다는 단점을 가지고 있다.
플라스틱 재질의 소초를 사용할 경우에는 조소가 원활히 이루어지지 않아, 소초광에 hot melt spray 방식이나 또는 롤러를 이용하여 밀납을 소초 표면에 코팅하는 과정이 필요하다. 고분자 물질은 우수한 기계적 물성 및 낮은 무게와 저렴한 생산비용의 장점을 가짐으로써 현대사회에 있어서 가장 많이 사용되는 재료이다. 그러나 양적 수요와 함께 환경오염 문제가 대두되면서 재활용 및 재생성 플라스틱 재료의 연구 또한 매우 활발해지고 있다 (Yudakhina, 2022).
소초 제조용 재료는 폴리프로필렌 (polypropylene, PP)이 주로 사용된다. 폴리프로필렌은 열가소성 고분자의 일종으로 1954년에 발견된 이후 범용수지로서 넓은 분야에 이용되어 왔다. 폴리프로필렌은 낮은 밀도와 우수한 내열성 및 기계적 강도를 가지고 무독성, 내약품성 및 전기절연성으로 인해 식품 포장, 포장 용기 등의 상업적 용도로 널리 사용되며, 관련 제품들은 주로 금형 사출 방식으로 생산되고 있다 (Maddah, 2016).
본 연구는 소초의 제조 목적으로 벌 친화성을 높이기 위하여 밀납을 첨가한 PP 복합재료를 개발하는 동시에, 기존 사출 제조 방식의 단일 생산 시에 부담이 되는 금형제작 비용을 절감하고자 우선 3D 프린팅 방식의 소초 제조를 수행하였다 (Abdelrazeq et al., 2019). 열가소성 고분자는 상대적으로 낮은 기계적 강도를 보완하기 위해 충진재를 첨가하여 복합화하며, 여기서도 밀납 첨가로 인한 기계적 강도의 저하를 상쇄시키기 위해 이러한 충진재를 사용하는 것이 고려되었다 (Zhang et al., 2016; Graziano et al., 2019). 전형적인 충진재로서 활석 (talc), 탄산칼슘 (calcium carbonate), 유리섬유 등이 있으며, 친환경성을 고려하여 WPC (wood-plastic composite)가 기계적 물성 강화에 사용된다 (Lapcik et al., 2008; Adhikari et al., 2012; Sathishkumar et al., 2014; Nikmatin et al., 2017; Lee et al., 2020; Mark et al., 2020; Parres et al., 2020).
ISO/ASTM 52900에 따르면, 3D 프린팅 기술은 적층제조 (additive manufacturng, AM) 혹은 쾌속 조형 (rapid prototyping, RP)으로 명명된다. 1984년 Chuck hull에 의해 개발된 광경화성 수지 조형 방식의 SLA 프린터를 시초로, Stratasys에 의해 주도적으로 개발된 적층 조형 방식을 사용하여 디지털 모델을 금형 없이 바로 출력할 수 있다 (Wahab et al., 2013; Perez et al., 2020; Kristiawan et al., 2021). 금형이 별도로 요구되지 않기에 사출성형에 비해 개당 생산단가가 저렴하고 공정이 간소하여 적용 가능한 재료가 다양하다는 장점을 가짐으로써, 3D 프린터는 항공, 자동차, 의료, 건설 등 다양한 분야로 확대 적용되어 왔다 (Doshi et al., 2022).
FDM (fused deposition modeling)은 가장 대중화된 3D 프린팅 방식으로 SLS (selective laser sintering) 및 SLA (stereo lithography apparatus)와 달리 고분자 필라멘트를 압출하여 적층시키는 방식이다. 다른 방식에 비해 출력속도가 빠르고 메커니즘이 간단하여 접근성 측면에서 가장 대중화된 방식이긴 하지만 사용 가능한 고분자 재료가 제한적인 단점이 있다 (Sanatgar et al., 2017; Mazzanti et al., 2019).
고분자 재료가 3D 프린팅 기술에 적용될 경우 얻을 수 있는 이점은 매우 크나, FDM 방식의 3D 프린팅 기술을 적용하기 위해서는 출력 시 결정성 고분자의 재결정화 과정에 의한 형상 변형 및 수축 현상을 개선하여야 한다. 본 연구에서는 homo PP에 밀납, 활석, 목분 (wood flour, WPC) 및 상용화제 (compatibilizer, cpt)를 첨가하여 PP 복합재료를 만든 후, 필라멘트 압출기를 사용하여 1.75 mm 두께의 필라멘트를 제조하고, 이어서 FDM 방식의 3D 프린터를 이용하여 조형물을 출력시켰다. 이에 따른 최적 출력조건을 얻기 위하여 PP/beeswax 복합재료 시편의 기계적 및 열적 물성을 측정하고 첨가제 비율에 따른 3D 프린팅 과정에서의 변형률 및 형태 안정성을 평가하였다.
실험 방법
1. 실험 재료
복합재료의 주 원료인 폴리프로필렌은 GS칼텍스사의 H710을 사용하였다. WPC는 60% M/B (masterbatch)가 이용되었으며, talc는 코츠원사 (KOCHONE Co.)의 KC-2000이 그리고 상용화제로는 IruChem Co.의 TPX-1380이 사용되었다.
2. 재료 조성
PP 복합재료 원료의 구성성분 및 배합비를 Table 1에 나타내었다. 복합재료의 기지재는 homo PP, 충진재로는 목분 및 활석, 밀납, 상용화제가 사용되었다. 목분과 활석은 복합재료의 기계적 강도 향상을 위해 각각 10 wt%가 첨가되었으며, 밀납의 조성 유무에 따른 물성 변화를 비교하기 위해 밀납 미첨가 및 10 wt% 첨가 복합재료를 각각 컴파운딩하였다. 또한 상용화제 조성 유무에 따른 기계적 강도 및 수축률 변화를 비교하기 위해 2 wt%를 첨가한 시료를 컴파운딩하였다.
PP 복합재료는 FILIBOT H303 (Fordentech Co., Korea)을 사용하여 1.75 mm 두께의 필라멘트로 압출되었다. 압출 과정에서 압출기 온도는 175℃로 유지하여 압출 및 수냉 공정을 거친 후 권선되었다. Fig. 1 및 2는 원료의 컴파운딩 공정과 필라멘트의 압출 과정을 각각 보여주고 있다.
3. 3D 프린팅
PP 복합재료의 3D 프린팅 적합성 여부를 확인하기 위하여 dimensional accuracy, deformation rate 및 warp-index의 3가지 측정 방식을 통해 출력물의 형태변형 정도를 비교하였다. PP 복합재료 필라멘트는 출력을 위해 노즐 두께 0.5 mm의 FDM 방식의 프린터 (상상이상 3D프린팅, Core-XY 500, Korea)에 적용되었다. PP는 소재 특성상 다른 물질과의 접착력이 매우 약하므로, 베드와 첫 레이어 간의 접착력 향상을 위해 출력베드 표면은 PP 성분의 테이프로 처리하였다. 출력된 시편은 상온에서 24 h 동안 유지하여 안정화시킨 후 측정이 이루어졌다. 3D 프린팅은 노즐온도 230℃, 베드온도 80℃, 출력속도 30 mm/sec의 기본 조건에서 행하여졌으며, 레이어 두께 0.2 mm 및 infill density 20%로 설정되었다.
Dimensional accuracy는 Fig. 3과 같은 20×20×20 mm의 XYZ calibration cube 모델을 출력한 후, 식 1에 따라 오차율을 계산하였다 (Shahrubudina et al., 2019).
(1) |
Deformation rate는 ASTM D955-21에 의거 60×60×2 mm 형상의 정사각형 평판 (square plaque)을 출력하였으며, 시편은 바닥-표면 간의 거리를 측정하여 형태변형을 평가하였다 (Dong et al., 2019). 3D 프린팅의 최적 조건을 조사하기 위해 PP/beeswax 복합재료는 노즐온도, 출력속도 및 베드온도의 변화에 따라 출력된 후, 실험 변수에 따른 변형도를 비교하었다.
Warp 측정을 위해서는 1 mm 넓이의 레이어를 10 mm 간격으로 표시, 적층하여 커튼 형태로 출력되었다 (Fig. 4). 이 출력물을 평면 스캔하여 레이어의 굴곡을 측정함으로써, warp-index가 식 2에 의해 얻어졌다.
(2) |
4. 특성 분석
PP 복합재료의 재료적 특성을 확인하기 위해 기계적 물성 및 열적 물성을 측정하였다. 기계적 물성을 측정하기 위해 만능재료시험기 (ARG-X STD, Instron)를 사용하여 인장시험 및 3점 굽힘시험을 수행하였다. 인장은 50 mm/min 조건으로 시험하여 인장강도, 신률 및 탄성계수를 측정하고, 3점 굽힘은 10 mm/min 조건으로 시험하여 굴곡강도 및 굴곡탄성률을 측정하였다. 충격강도는 Izod 충격시험기 (IT 504 Impact Tester, Tinius Olsen)를 사용하여 상온 (23℃) 및 -10℃에서 각각 측정하였다. 시험 과정에서 인장은 ASTM D638, 굴곡은 ASTM D790 그리고 Izod 충격은 ASTM D256 기준에 따라 수행되었다.
PP 복합재료의 결정화도 분석을 위해 X-ray 회절분석법 (XRD, Malvern Panalytical)을 이용하여 10~50° 범위의 회절 데이터를 측정하여 결정화도를 분석하였다.
열적 특성 평가를 위해 시차주사열량계 (DSC 4000, PerkinElmer)를 사용하여 질소 기류하에 승온속도 10℃/min에서 30~300℃의 조건으로 측정하였다.
PP 복합재료의 충진재 구성에 따른 형태학적 특성은 Izod 충격강도 시편을 10 keV 조건에서 200 및 4000배율로, 그 파단면을 주사전자현미경 (scanning electron microscope, SEM)으로 관찰함으로써 확인하였다.
결과 및 고찰
1. 기계적 성질
충진재에 따른 PP의 기계적 강도를 분석하기 위해 인장시험, 3점 굽힘시험 및 Izod 충격시험을 수행하였다. 기계적 강도의 측정 결과, PP 복합재료의 인장강도와 신율은 서로 반대 성향을 나타내었다. Fig. 5(a)에서 인장강도는 밀납 첨가 시 강도가 떨어졌으나 신율은 Fig. 5(b)와 같이 크게 증가하였다. Fig. 5(c) 및 5(d)는 각각 굴곡강도 및 굴곡탄성률을 측정한 결과로서 밀납 첨가 시 굴곡 특성이 감소하였다. Fig. 5(e)는 Izod 충격강도 시험의 결과로 상온 조건인 23℃에서 PP compound의 수치는 46.3 J/m이고 PP/beeswax compound의 수치는 48.5 J/m으로 미세한 증가를 가지며, -10℃의 경우 PP compound는 33.3 J/m이고 PP/beeswax compound의 수치는 28.3 J/m를 나타냄으로써 저온에서 충격강도가 감소하는 모습을 보였다. 상용화제 첨가 유무에 따른 PP 복합재료의 기계적 물성 비교 시, 상용화제가 첨가된 시편은 굴곡탄성률을 제외하고 기계적 강도가 모두 저하되었다.
2. Morphology
PP compound의 충진재에 따른 형상 변화를 관찰하기 위해 SEM을 사용하여 분석한 시편의 파단면을 Fig. 6에 나타내었다.

Cross-section of PP composite materials. (a) PP compound 200 x, (b) PP/beeswax 200 x, (c) PP/beeswax/compatibilizer 200 x, (d) PP compound 4.0 kx, (e) PP/beeswax 4.0 kx, and (f) PP/beeswax/compatibilizer 4.0 kx.
SEM 분석 결과 세 종류의 시료는 Fig. 6에 나타난 바와 같이 활석 및 목분이 모두 관찰되었다. 활석의 경우는 작은 알갱이 형태이나 목분 입자는 평판상으로 나타났다. 4000배율로 관찰한 결과, 상용화제의 첨가는 얇은 섬유상 형태로 분산된 것이 Fig. 6(f)에서 확인되었다. Fig. 6(b) 및 6(c)에서 관찰되는 약 150 μm 크기의 입자체는 밀납으로 보여진다.
3. 결정성
PP 복합재료의 첨가제 비율에 따른 결정성 변화를 비교하기 위해 XRD를 사용하여 결정화도를 측정한 결과를 Fig. 7에 나타내었다. 결정화도 (relative crystalinity)는 XRD pattern에서 baseline-peak의 전체 면적 (global area)으로 나누어진 crystalline peak의 내부 면적 (peak area)에 대한 백분율로 정의된다.
XRD 측정 결과 PP 14°, 17°, 28°에서 뚜렷한 피크강도가 나타났으며, 밀납 첨가 시 28° 부근에서 피크의 강도가 크게 증가하는 것이 관찰되었다. 밀납 첨가 PP compound의 결정화도는 91.0%에서 84.8%로 감소하였으며, 이는 폴리프로필렌의 비율 감소에 기인할 것이다. 상용화제 첨가 시 시료의 결정화도는 미세하게 증가하나 복합재료의 결정성에는 큰 영향을 주지 않는 것으로 판단된다. 고분자의 결정화도가 증가할 경우, 재결정화에 의해 3D 프린팅 출력물의 수축률이 증가함으로써 출력물의 형태 변형에 영향이 있을 것으로 예상된다.
4. 열적 특성
DSC 분석의 결과 Fig. 8에서와 같이 세 가지 시료가 모두 165℃에서 용융피크가 관찰되었다. PP compound, PP/beeswax 및 PP/beeswax/compatibilizer 비교 시, beeswax 및 상용화제의 첨가로 인하여 복합재료의 용융피크는 오른쪽으로 이동하는 동시에 피크의 강도가 점차 약해지고 범위가 넓어지는 경향을 보였다.
5. 3D 프린팅 최적화
PP 복합재료의 3D 프린팅 성능을 분석하기 위해 PP 복합재료를 FDM 3D 프린터를 사용하여 출력하였다. 출력 시편은 24 h 동안 상온 보관되었으며, Fig. 9에서와 같이 수축 현상에 의한 형태 변형이 관찰되었다. Dimensional accuracy의 측정 결과, Fig. 10과 같이 밀납 첨가 시 PP 복합재료의 dimensional accuracy 수치가 감소하였다. 시편의 출력 방향에 따른 수치는 Z 방향의 값이 가장 높게 나왔는데, 이는 적층된 레이어 간의 이격에 따른 변수에서 비롯된다. 상용화제의 첨가 유무에 따른 dimensional accuracy를 비교할 경우, 상용화제 첨가 시 X 및 Y 방향의 수치는 감소하여 설정된 형태에 근접하게 출력되었으나 Z 방향에서 상대적으로 높은 수치를 보여주었다. Fig. 11은 warp-index 측정을 통한 레이어 길이당 곡률 측정의 결과로 PP compound는 20~40 mm 길이의 레이어에서 warpage가 뚜렷이 큰 수치를 보였다.
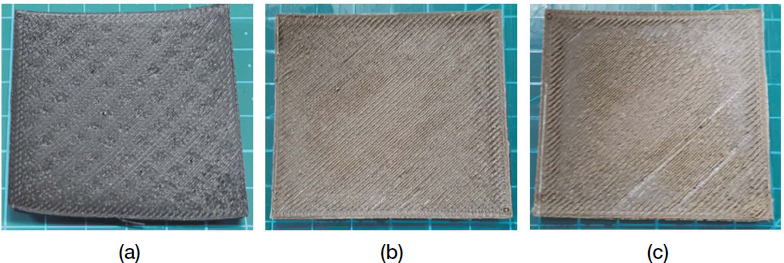
Deformation of square flat PP composites. (a) PP compound, (b) PP/beeswax, and (c) PP/beeswax/compatibilizer.
PP/beeswax compound는 30 mm 그리고 PP/beeswax/compatibilizer는 10 mm 길이의 레이어에서 변형이 크게 일어났으며, 두 시료 모두 50 mm 길이의 레이어에서 가장 낮은 warpage를 보였다. Beeswax가 첨가된 복합재료는 dimensional accuracy와 마찬가지로 warp-index를 크게 감소시켰다. PP/beeswax 및 PP/beeswax/compatibilizer의 compounds에 대한 warp-index의 평균치를 비교하였을 때, 곡률이 미세하게 증가하였으나 큰 영향을 주지 않는 것으로 판단된다.
Deformation rate의 측정 결과, PP 복합재료의 변형률은 밀납 첨가 시 감소하는 것으로 나타났다 (Fig. 12). 또한 상용화제의 첨가 유무에 따른 변형률 변화는 미첨가 시료보다 변형을 더 감소시켜 수축 현상을 개선하는 효과를 보였다. 밀납 및 상용화제를 동시에 첨가한 결과, 평균 변형률은 4.1에서 3.3으로, 최대 변형률은 6.7에서 3.9까지 감소하였다.
3D 프린팅의 최적화 조건을 선정하기 위해 노즐온도, 표면온도 및 출력속도를 측정 변수로 설정하였으며, 이러한 프린팅 조건에 따라 얻어진 deformation rate를 Fig. 13에 나타내었다. 기본조건은 노즐온도 240℃, 표면온도 90℃, 출력속도 30 mm/sec로서 각각의 변수에 따라 노즐온도 230, 240 및 250℃, 표면온도 80, 90 및 100℃ 그리고 출력속도 20, 30 및 40 mm/sec로 설정을 변경하여 프린팅을 수행하였다. 그 결과, 노즐온도와 표면온도 변화에 따른 변형은 일정한 경향성을 보이지 않았으나 각각 240℃ 및 90℃의 조건에서 변형이 가장 적게 일어났다. 출력속도의 경우 속도가 빠를수록 변형이 증가하는 것이 확인되었다. 이는 출력속도가 증가할수록 출력물의 표면접착력이 상대적으로 감소함에 따른 것으로 사료된다. 출력속도 저하 (40 → 20 mm/sec)에 따른 deformation rate의 변화는 3.6에서 3.1까지 감소하는 효과를 보였다.
결 론
폴리프로필렌에 밀납 및 충진제를 첨가한 복합재료의 기계적 물성 측정 및 3D 프린팅 최적화 시험을 수행한 결과, 밀납 첨가는 복합재료의 기계적 강도를 감소시키나 연신율은 큰 폭으로 증가하는 것으로 나타났다. 밀납 10 wt%가 첨가된 복합재료는 기존 PP 복합재료와의 비교 시 연신율이 120% 증가한 반면 인장강도는 15% 감소하였고, 상온 환경에서 Izod의 충격강도를 증가시키는 효과를 보여주었다. 상용화제 2 wt%의 첨가는 복합재료의 전반적인 기계적 물성을 소량 감소시켰으나, 3D 프린팅 시험 결과와 비교했을 때 복합재료의 수축 현상을 감소시켜 형태 안정성을 증가시키는 효과를 나타내었다. 또한 밀납이 10 wt%까지 첨가된 PP 복합물이 필라멘트 압출 및 3D 프린팅을 가능하게 하였다. Dimensional accuracy, deformation rate 및 warp-index의 세 가지 측정법을 통해 수축 현상에 의한 형태 변형률을 조사한 결과, 밀납 및 상용화제를 함께 첨가 시 수축이 최소화됨을 확인하였다. 출력 변수에 의한 프린팅 최적화의 경우, 노즐온도 및 베드온도는 뚜렷한 경향성을 나타내지 않았으나 출력속도는 낮을수록 형태변형이 적어지는 것을 확인하였다. XRD 분석을 통한 결정화도 측정 결과와의 비교에서 결정화도의 감소로 인해 수축 현상은 줄어드나, 이에 따른 기계적 강도의 감소가 동시에 수반되었다.
적 요
농가의 인력부족 현상과 친환경 소재의 사용 경향을 전망해 보면, 벌집에 대하여 반영구적인 플라스틱 소초광의 필요성과 생산성 향상 방안이 절실히 요구되고 있다. 꿀벌과 친화력이 있고 높은 조소율을 가진 벌집판을 제조하기 위한 재료로서 친환경성 고분자인 폴리프로필렌 (Polypopylene, PP)의 복합물을 선정하였다. 또한 꿀벌의 조소성을 더욱 향상시키기 위한 방법으로 성형된 벌집판 표면에 밀납 코팅을 하는 것이 일반적이나, 이 경우 간혹 소초의 기공이 막히는 문제점이 있으므로 PP 소재에 밀납 성분을 직접 첨가시켜 벌집판을 제조할 수 있다. 플라스틱 벌집판은 상업적으로 금형을 이용한 사출 또는 압축성형법으로 대부분 생산된다. 본 연구에서 3D 프린팅 방법으로 플라스틱 벌집판을 제조하기 위하여, PP 수지에 밀납, 활석, 목분 및 상용화제를 첨가시킨 조성물이 펠렛 성형되고 1.75 mm 두께의 필라멘트로 압출되었다. 3D 프린팅 변수들은 노즐온도, 베드온도 및 출력속도로 설정하였으며, 출력물의 특성은 dimensional accuracy, deformation rate 및 warp-index를 비교함으로써 평가되었다. PP 복합재의 플라스틱 벌집판은 재생 가능하고 내구성이 뛰어나, 기존 일회용 밀납 벌집의 사용으로 인한 환경오염을 방지할 뿐만 아니라 양봉산업의 경제성 향상에도 크게 기여할 수 있을 것이다.
Acknowledgments
본 연구는 농촌진흥청 농업정책지원기술개발사업 (과제번호 RS-2021-RD010194)으로 수행하였음.
References
-
Abdelrazeq, H., P. Sobolčiak, M. A. A. Al-Maadeed, M. Ouederni and I. Krupa. 2019. Recycled Polyethylene/Paraffin Wax/Expanded Graphite Based Heat Absorbers for Thermal Energy Storage: An Artificial Aging Study. Molecules 24(7): 1217.
[https://doi.org/10.3390/molecules24071217]
-
Abdikheibari, S., R. Parvizi, M. H. Moayed, S. M. Zebarjad and S. A. Sajjadi. 2015. Beeswax-colophony blend: A novel green organic coating for protection of steel drinking water storage tanks. Metals 5(3): 1645-1664.
[https://doi.org/10.3390/met5031645]
-
Adhikari, R., N. L. Bhandari, H. H. Le, S. Henning, H. J. Radusch, G. H. Michler and J. M. Saiter. 2012. Thermal, mechanical and morphological behavior of poly (propylene)/wood flour composites. Macromol. Symp. 315: 24-29.
[https://doi.org/10.1002/masy.201250503]
- Dong, M., S. Zhang, G. Dali and C. Baige. 2019. The study on polypropylene applied in fused deposition modeling. AIP Conf. Proc. 2065: 030059-1-030059-5.
-
Doshi, M., A. Mahale, S. K. Singh and S. Deshmukh. 2022. Printing parameters and materials affecting mechanical properties of FDM-3D printed Parts: Perspective and prospects. Mater. Today: Proceedings 56(5): 2269-2275.
[https://doi.org/10.1016/j.matpr.2021.10.003]
-
Fratini, F., G. Cilia, B. Turchi and A. Felicioli. 2016. Beeswax: A minoreview of its antimicrobial activity and its application in medicine. Asian Pac. J. Trop. Med. 9(9): 839-843.
[https://doi.org/10.1016/j.apjtm.2016.07.003]
-
Graziano, A., S. Jaffer and M. Sain. 2019. Review on modification strategies of polyethylene/polypropylene immiscible thermoplastic polymer blends for enhancing their mechanical behavior. J. Elastomers Plast. 51(4): 291-336.
[https://doi.org/10.1177/0095244318783806]
-
Kristiawan, R. B., F. Imaduddin, D. Ariawan, Z. Ubaidillah and Z. Arifin. 2021. A review on the fused deposition modeling (FDM) 3D printing: Filament processing, materials, and printing parameters. De Gruyter 11: 639-649.
[https://doi.org/10.1515/eng-2021-0063]
-
Lapcik Jr., L., P. Jindrova, B. Lapcikova, R. Tamblyn, R. Greenwood and N, Rowson. 2008. Effect of the talc filler content on the mechanical properties of polypropylene composites. J. Appl. Polym. Sci. 110(5): 2742-2747.
[https://doi.org/10.1002/app.28797]
-
Lee, C., M. M. Pang and S. C. Koay. 2020. Talc filled polylactic-acid biobased polymer composites: tensile, thermal and morphological properties. SN Appl. Sci. 2: 354.
[https://doi.org/10.1007/s42452-020-2172-y]
-
Li, D., J. Zhang, W. C. Xu and Y. Fu. 2016. Effect of SiO2/EVA on the mechanical properties, permeability, and residual solvent of polypropylene packaging films. Polym. Compos. 37(1): 101-107.
[https://doi.org/10.1002/pc.23159]
- Maddah, H. A. 2016. Polypropylene as a promising plastic: A review. Am. J. Polym. Sci. 6 (1): 1-11.
-
Mark, U. C., I. C. Madufor, H. C. Obasi and U. Mark. 2020. Influence of filler loading on the mechanical and morphological properties of carbonized coconut shell particles reinforced polypropylene composites. J. Compos. Mater. 54(3): 397-407.
[https://doi.org/10.1177/0021998319856070]
-
Mazzanti, V., L. Malagutti and F. Mollica, 2019. FDM 3D printing of polymers containing natural fillers: A review of their mechanical properties. Polymers 11(7): 1094.
[https://doi.org/10.3390/polym11071094]
-
Nikmatin, S., A. Syafiuddin, A. Kueh and A. Maddu. 2017. Physical, thermal, and mechanical properties of polypropylene composites filled with rattan nanoparticles. J. Appl. 15(4): 386-395.
[https://doi.org/10.1016/j.jart.2017.03.008]
-
Parres, F., M. A. Peydro, D. Juarez, M. P. Arrieta and M. Aldas. 2020. Study of the Properties of a Biodegradable Polymer Filled with Different Wood Flour Particles. Polymers 12(12): 2974.
[https://doi.org/10.3390/polym12122974]
-
Perez, M., D. Carou, E. M. Rubio and T. Teti. 2020. Current Advances in Additive Manufacturing. Procedia CIRP 88: 439-444.
[https://doi.org/10.1016/j.procir.2020.05.076]
- Sanatgar, R. H., C. Campagne and V. Nierstrasz. 2017. Investigation of the adhesion properties of direct 3D printing of polymers and nanocomposites on textiles: Effect of FDM printing process parameters. Appl. Surf. Sci. 50: 2269-2275.
-
Sathishkumar, T. P., S. Satheeshkumar and J. Naveen. 2014. Glass fiber-reinforced polymer composites - a review. J. Reinf. Plast. Compos. 33(13): 1258-1275.
[https://doi.org/10.1177/0731684414530790]
-
Shahrubudina, N., T. C. Lee and R. Ramlana. 2019. An Overview on 3D Printing Technology: Technological, Materials, and Applications. Procedia Manuf. 35: 1286-1296.
[https://doi.org/10.1016/j.promfg.2019.06.089]
-
Wahab, M. S., A. Wagiman and M. Ibrahim. 2013. Development of wood-based composites material for 3D printing process. Appl. Mech. Mater. 315: 987-991.
[https://doi.org/10.4028/www.scientific.net/AMM.315.987]
-
Yudakhina, M. A. 2022. Ecological features and the influence of hive materials on the viability of bee colonies in Eastern Siberia. IOP Conf. Ser.: Earth Environ. 981.
[https://doi.org/10.1088/1755-1315/981/4/042083]