
Population Modeling of Bombus hypocrita sapporoensis under Climate Change Scenarios
Abstract
The study on the impacts of climate change on Bombus hypocrita sapporoensis highlights how rising temperatures, precipitation, and humidity influence the distribution and activity of this bumblebee population across South Korea. Certain regions, such as Gangwon and Jeju, are projected to be more favorable for B. h. sapporoensis during the summer, while areas like Jeollanam-do and Gyeongsangnam-do may experience increased temperature variability that could negatively impact winter survival. Climate projections indicate that rising temperatures may increase summer activity and population growth in some areas. However, it could also reduce the overall population in other regions due to limited winter survival, with significant regional differences in habitat suitability. The model used in this study effectively predicted these changes, demonstrating strong accuracy by incorporating key climatic variables and simulating different scenarios, thereby providing valuable insights into future distribution patterns and challenges for B. h. sapporoensis in response to climate change.
Keywords:
Bombus hypocrita sapporoensis, Climate change, Species distribution modeling, Habitat suitability, South KoreaINTRODUCTION
Bombus hypocrita sapporoensis is an effective pollinator with significant ecological and agricultural value, particularly in native ecosystems and economically important crops (Sirois-Delisle and Kerr, 2018; Takeuchi et al., 2018). As a key pollinator species, B. h. sapporoensis plays a vital role in maintaining biodiversity and providing essential ecosystem services, making its conservation highly important (Nagamitsu et al., 2005). In South Korea, B. h. sapporoensis, along with other bumblebee species like Bombus, is regarded as a valuable genetic resource due to its role as a vital pollinator. However, the impacts of climate change pose a significant threat to the spatial distribution and population dynamics of this species, which must be addressed to ensure its long-term survival (Kwon, 2008). Recent declines in global pollinator populations have raised serious concerns regarding the health of ecosystems and agricultural productivity. B. h. sapporoensis, in particular, provides unique and irreplaceable ecological services in both wild and cultivated environments, making it a crucial species for comprehensive study and targeted conservation efforts (Nagamitsu et al., 2005; Tsuchida et al., 2019).
Existing studies on B. h. sapporoensis populations have primarily focused on demographic characteristics, habitat preferences, and assessments of threats such as habitat loss and pesticide exposure (Barbieri et al., 2010; Yokoyama and Inoue, 2010). While these studies have provided valuable insights, the potential impacts of climate change on the population dynamics and geographic distribution of this species remain relatively unexplored (Potts et al., 2010; Banks et al., 2017). Existing research indicates that bumblebees may be more resilient to fluctuating weather conditions compared to honeybees (Karbassioon et al., 2023). Their adaptability to lower temperatures, higher humidity, and stronger winds suggests bumblebees could be important for pollination as the climate changes (Clarke and Robert, 2018). According to a study on wild bee species in northwestern Iran, climate change is projected to significantly influence these populations̓ geographic distribution (Rahimi et al., 2021). Specifically, rising temperatures are anticipated to expand the potential range for these bee species, leading to a northward shift in their distributions (Ghassemi-Khademi et al., 2021). Although studies on other bumblebee species have provided insights into their potential resilience to climate change, the specific impacts on the population dynamics and geographic distribution of B. h. sapporoensis remain largely unexplored in South Korea. This study aims to address this critical research gap by developing a population model for B. h. sapporoensis that incorporates the potential impacts of climate change.
In researching the impact of climate change on B. h. sapporoensis in South Korea, the use of climate indices, such as climate days, provides significant advantages over individual weather variables. Climate indices offer a comprehensive view by integrating multiple weather factors, allowing for a clearer identification of trends and patterns that might not be visible when examining variables separately (Change, 2023). This approach is particularly effective for assessing the frequency and intensity of extreme weather events, which are crucial for understanding the impacts on bee populations. By simplifying complex climate data into indices, researchers can more effectively communicate findings to policymakers and the public, facilitating informed decision-making regarding adaptation and mitigation strategies (Weiskopf et al., 2020; Rahimi and Jung, 2024).
The high-emission SSP 5.85 scenario, which represents limited mitigation efforts, provides a valuable framework for evaluating the potential impacts of climate change on ecosystems (An et al., 2022). This scenario allows for a comprehensive assessment of the potential effects of climate change on B. h. sapporoensis populations. Modeling Bumble bee populations under the SSP 5.85 scenario is crucial for predicting potential population declines, shifts in distribution, and identifying critical conservation needs (Banks et al., 2017). This information will help pinpoint vital habitats and support formulating mitigation strategies to protect pollinator health and biodiversity under future climate conditions.
The primary objective of this study is to develop a population model for B. h. sapporoensis using climate indices based on climate days. The objectives of this study are twofold: first, to develop a comprehensive population model for B. h. sapporoensis that incorporates climate indices, and second, to assess the potential impacts of various climate change scenarios on the population dynamics and geographic distribution of this vital pollinator species.
This study aims to provide a comprehensive understanding of how environmental factors, especially those influenced by climate change, impact the behavior, survival, and geographic distribution of B. h. sapporoensis.
METHODS
This study employs a comprehensive methodology that integrates observational meteorological data and climate scenario projections to assess the impact of weather conditions on the activity and survival of Bombus hypocrita sapporoensis. By combining field observations, advanced spatial interpolation techniques, and statistical modeling, we aim to provide a detailed understanding of how environmental factors influence bee behavior under both current and future climate scenarios. This approach allows us to capture the complex interactions between bees and their environment, accounting for critical factors such as temperature, precipitation, and humidity, which are essential for understanding bumblebee population dynamics. Through the use of advanced modeling and interpolation methods, we can generate high-resolution spatial data to analyze the impacts of climate change on B. h. sapporoensis habitats and distribution across the study region.
1. Meteorological data and climate scenario projections
The meteorological data used in this study were obtained from multiple reliable sources, ensuring a robust dataset that captures both general and region-specific weather conditions. Observational data were primarily sourced from the Korean Meteorological Administration, which provides data from the Automatic Surface Observing System (ASOS) and Automatic Weather Stations (AWS). Additionally, the Korea Forest Service contributed data from Automatic Mountain Meteorology Observation Stations (AMOS), which specifically monitor areas above 200 meters in altitude (Jang et al., 2017). This combination of data sources allowed us to account for the diverse weather conditions across both lowland and mountainous areas, given Korea̓s complex topography (Kim et al., 2021a). The dataset included daily measurements of maximum, minimum, and average temperatures, as well as precipitation. This comprehensive meteorological data encompassed a wide range of variables, including not only temperature and precipitation, but also relative humidity and wind speed. By incorporating these additional weather parameters, the study was able to gain a more nuanced understanding of the environmental factors that influence the activity and survival of B. h. sapporoensis in the region.
In addition to observational data, the study also utilized future climate scenarios modeled using the GFDL-ESM2G Earth System Model. This model incorporates a detailed carbon system formulation, allowing it to represent the complex interactions between the climate and the global carbon cycle under anthropogenic influences (Dunne et al., 2012). The GFDL-ESM2G model employs generalized ocean layer dynamics and interactive carbon dynamics, enabling more accurate simulation of coupled climate-carbon responses, particularly with respect to ocean ventilation and nutrient cycling, which significantly affect carbon uptake and the strength of the biological carbon pump. The model also incorporates dynamic vegetation, which allows for a more comprehensive representation of Earth system processes and their feedback mechanisms (Wilson et al., 2022).
The study utilized the representative concentration pathway SSP 5.85, which outlines a high-emission scenario with limited mitigation efforts (Rao et al., 2016). These SSP scenarios provided projected daily maximum, minimum, and average temperatures, as well as daily precipitation. Diurnal temperature ranges were derived by subtracting daily minimum temperatures from daily maximum temperatures. Both observational and climate scenario datasets were rigorously preprocessed, including handling missing values, normalization, and scaling of variables to ensure consistency and data quality for further analysis.
2. Spatial interpolation and climate raster generation
To generate continuous spatial data across the study area, we used two interpolation techniques. Inverse Distance Weighting estimated unknown values by calculating a weighted average of nearby known data points, with closer points having greater influence. However, IDW does not account for the significant temperature lapse rate effects in mountainous regions, which can lead to biased temperature estimates, especially at higher elevations. Adjusting temperature lapse rates was crucial to accurately estimate temperatures, especially in regions with significant elevation variations. Previous research has shown that the spatial variability of temperature in mountainous regions is strongly influenced by elevation, necessitating the consideration of lapse rates to accurately reflect temperature gradients (Tennakoon et al., 2024). This approach is especially effective when the available meteorological station network is dense, as it ensures that local temperature variations are well represented.
The Inverse Distance Weighting formula used is as follows:
- Where:
- • z=the estimated value at the point of interest
- • wi=the weight assigned to each known data point i
- • vi=the known value at data point i
- • di=the distance between the point of interest and each known data point i. The weight, wi, is typically determined by the inverse of the distance di raised to a power parameter, which controls the influence of distance. This formula allows for the interpolation of climatic variables across the study area, capturing the spatial variability in temperature and precipitation patterns.
This formula allows for the interpolation of climatic variables across the study area, capturing the spatial variability in temperature and precipitation patterns.
To generate continuous spatial data across the study area, the MK-PRISM regression approach was utilized, which accounts for the influence of topographic characteristics, including elevation, slope, and aspect, on the spatial distribution of climatic variables (Kim, 2014). This method enables the incorporation of the effects of these terrain characteristics on the spatial patterns of temperature and precipitation. By integrating the MK-PRISM regression technique, we generated high-resolution climate rasters that capture the complex spatial patterns of temperature and precipitation across the study region, including the nuanced effects of the mountainous terrain. The MK-PRISM regression equation used in this study takes the following form:
- Where:
- • Yi represents the estimated climate variable at location i,
- • β0, β1, β2, and β3 are regression coefficients that capture the influence of each topographic variable,
- • Elevi, Slopei, and Aspecti denote the elevation, slope, and aspect at location i, respectively,
- • and єi is the error term.
This approach is particularly well-suited for capturing the variability in precipitation that arises from Korea̓s mountainous terrain, as it incorporates the effects of topographic features such as elevation, slope, and aspect on the spatial distribution of climatic variables.
To evaluate the impacts of climate change on habitat suitability and population dynamics of B. h. sapporoensis, a comprehensive suite of seasonal weather indices was developed to capture the key environmental factors influencing this bumblebee species.
The optimal weather conditions for bees capture the influence of factors like temperature and humidity on their behavior across different seasons. For instance, conditions deemed ideal for B. h. sapporoensis were days with an average temperature between 10°C and 30°C, a minimum of 7°C, and a maximum not exceeding 34°C (Kwon and Saeed, 2003). These parameters are known to maximize foraging efficiency and colony growth (Nagamitsu et al., 2005). In contrast, extremely hot summer days with an average above 34°C can hinder thermoregulation and foraging, especially for heat-sensitive bumblebees (Papanikolaou et al., 2017).
Cold winter conditions, defined as days with an average temperature below 7°C, can limit bee foraging activity and increase overwintering mortality due to the higher energy demands needed to maintain hive temperatures (Jaboor et al., 2022). Similarly, spring dryness, characterized by relative humidity below 0.5, can reduce nectar availability, negatively impacting foraging behavior and colony health. Additionally, winter mild-temperature days, with average temperatures between 7-10°C, and winter warm days, with average temperatures above 10°C, were also tracked, as unseasonably warm temperatures can lead to premature activity, depleting critical energy reserves and threatening colony survival over the long winter months (Karbassioon et al., 2023). Based on the information from the literature, the following seasonal weather indices were generated to capture the weather conditions relevant to the activity of B. h. sapporoensis. The formulas are as follows:
- • Optimal Spring Weather Days (WF1) Conditions: Average temperature between 10°C and 30°C, minimum temperature of 7°C or above, and maximum temperature not exceeding 34°C Analysis Period: March 1 to May 31 each year
- • Hot Spring Weather Days (WF2) Conditions: Average temperature exceeding 34°C Analysis Period: March 1 to May 31 each year
- • Cold Spring Weather Days (WF3) Conditions: Average temperature below 7°C Analysis Period: March 1 to May 31 each year
- • Spring precipitation Weather Days (WF4) Conditions: Precipitation above 0.5 mm Analysis Period: March 1 to May 31 each year
- • Mild Winter Weather Days (WF5) Conditions: Average temperature between 0°C and 10°C Analysis Period: May 1 to February 28 follow year
- • Warm Winter Weather Days (WF6) Conditions: Average temperature exceeding 10°C Analysis Period: November 1 to February 28 follow year
These seasonal weather indices provide a comprehensive framework for quantifying the climatic conditions that impact the biology and population dynamics of B. h. sapporoensis, allowing us to assess the effects of climate change on this important bumblebee species.
The nest site observations were conducted over a comprehensive 10-year period from 2014 to 2023. The survey encompassed a diverse range of regions throughout the country, with a focus on mountainous areas and parks. This extensive timeframe allowed for a robust understanding of the temporal and spatial patterns in the nesting habits of B. h. sapporoensis. A consistent methodology was employed throughout the study to ensure data integrity and comparability. The fieldwork involved in-person visits to the survey sites, where direct observations and sample collections were carried out. The team focused on identifying and recording the precise geographical coordinates of B. h. sapporoensis nesting locations. Nesting sites were systematically identified, mapped, and documented to capture both the spatial distribution and environmental factors influencing their selection. The elevation of each location was recorded, providing insight into the potential effects of altitude on nest site preferences. The comprehensive dataset collected includes information on the nesting locations, such as latitude, longitude, altitude, the number of nests observed, and the specific collection dates. From 2014 to 2018, the data was used for the simulation model of B. h. sapporoensis, while data from 2019 to 2023 was utilized for validating the model. The survey covered a total of 130 sites across the country, primarily located in parks or along lake shores, as illustrated in Fig. 1. These variables are crucial for analyzing the ecological preferences and habitat requirements of B. h. sapporoensis. The detailed data will support further analyses aimed at understanding the species̓ nesting ecology, environmental correlates, and spatial distribution patterns across various altitudinal gradients and habitat types over the ten-year survey period.
A multiple linear regression model with backward elimination was employed to assess the relationship between seasonal weather metrics and bee survival. The model̓s independent variables were the previously described seasonal weather metrics, while the dependent variable was the overwintering mortality rate of bee colonies. Preprocessing the data was crucial to ensure the analysis̓s quality and reliability. This involved handling missing values through imputation or removal, based on the extent of missingness and its potential impact. Additionally, normalization and scaling were applied to align all variables on a comparable scale, mitigating biases in the regression model. Correlation analysis was also conducted to identify significant relationships between weather metrics and overwintering mortality, pinpointing the key factors influencing bee survival.
The multiple linear regression model was developed using data from 2014 to 2018 and then validated with data from 2019 to 2023, ensuring the predictions were grounded in real-world observations. This approach allowed for quantifying the impacts of various climatic factors on the survival and overwintering success of B. h. sapporoensis colonies, enabling more accurate projections of population dynamics under future climate change scenarios.
The model̓s performance was evaluated using the coefficient of determination,
The coefficient of determination, R2, is calculated as:
- R2=1-(SSres / SStot)
- where:
- • SSres is the sum of squares of residuals
- • SStot is the total sum of squares
This metric indicates the proportion of the variance in the dependent variable that is explained by the independent variables in the regression model.
RESULTS AND DISCUSSION
1. The regression model for the population dynamics of B. h. sapporoensis
The regression model for the population dynamics of Bombus hypocrita sapporoensis provides crucial insights into the environmental and geographical factors that influence the size of this species̓ colonies. The statistical results demonstrate the model̓s high significance, with an F-value of 33,605, indicating that the independent variables collectively play a substantial role in predicting the dependent variable-the number of individuals within the colonies (Table 1).
Furthermore, the model explains 91.8% of the variance in colony size, highlighting the robustness of the selected variables in understanding the ecological conditions governing the survival and proliferation of potentially limiting suitable habitat for B. h. sapporoensis. These environmental factors, including latitude, elevation, precipitation, and seasonal temperature patterns, are well captured in the model, and their combined effect is critical in predicting population trends (Table 2).
The high explanatory power of the model demonstrates the importance of these factors in shaping the population dynamics of B. h. sapporoensis. Based on the regression results, the final model equation for predicting the colony size of B. h. sapporoensis is expressed as:
- where:
- WF1=Optimal Spring Weather Days
- WF2=Hot Spring Weather Days
- WF3=Cold Spring Weather Days
- WF4=Spring precipitation Days
- WF5=Mild Winter Weather Days
- WF6=Warm Winter Weather Days
This model highlights the influence of various geographical and environmental factors. Among the variables examined, latitude has a marginal yet statistically significant positive impact on colony size. This suggests that colonies tend to perform slightly better at higher latitudes, potentially due to more favorable environmental conditions, such as cooler temperatures or reduced predator activity. Although the effect size is small, it underscores the potential role of geographic location in influencing colony success. Similarly, elevation exhibits a significant positive relationship with colony size, with a coefficient of 0.041. Higher-altitude regions appear to provide better conditions for B. h. sapporoensis, possibly due to factors such as cooler climates, lower human disturbance, or fewer competing species.
The model also identifies several environmental conditions that affect colony size. Precipitation days have a negative impact on colonies, with a coefficient of -1.206, indicating that increased rainfall disrupts foraging activities, leading to reduced resource accumulation and greater energy expenditure. These findings align with the known behavior of bumblebees, whose activity is often limited during rainy periods, resulting in lower food availability and compromised colony health. The importance of suitable winter conditions is also evident in the analysis. The number of Optimal Spring-to-Fall Weather Days positively influences colony size, suggesting that mild winter conditions enhance colony survival by allowing bees to maintain optimal cluster temperatures and conserve energy. Conversely, Hot Spring Weather Days negatively affect colony size, indicating that harsh spring conditions deplete energy reserves and increase mortality, underscoring the need for adequate winter shelter and supportive environments to ensure long-term survival. While the number of optimal Spring Weather days shows a positive but statistically insignificant effect, suggesting that favorable spring conditions alone may not be a decisive factor for colony size, the impact of extreme temperatures is more pronounced. Excessively high spring temperatures have a significant negative effect on colony size. This finding indicates that such conditions are detrimental to colony growth and survival, likely because they impair foraging efficiency and increase the metabolic stress on the bees. These results highlight the complex interplay between environmental factors and the biological needs of B. h. sapporoensis, where both extreme heat and precipitation act as significant stressors that limit colony success.
Several studies have explored the relationship between bumblebee populations and weather factors. Some study found that Bumble colonies tended to be larger and more abundant at higher elevations, potentially due to the cooler temperatures and reduced competition from other bee species in these areas (Yoon et al., 2002; Dolan et al., 2016). Additionally, a survey observed that B. h. sapporoensis nests were more frequently encountered in mountainous regions above 1,500 meters (Takeuchi et al., 2018; Tsuchida et al., 2019), suggesting the species̓ preference for higher-altitude habitats with more favorable environmental conditions. These findings align with the positive relationship between elevation and colony size observed in the regression model presented in this paper. However, the existing literature on the specific effects of other weather factors, such as precipitation and temperature extremes, on B. h. sapporoensis population dynamics is limited.
In comparison with previous studies on bumblebee populations, such as B. terrestris and B. impatiens, the results align with findings that extreme weather conditions, particularly high temperatures and heavy precipitation, play a significant role in colony success (Banks et al., 2017). Other studies have reported higher R2 values when focusing on smaller geographic regions or narrower environmental conditions, but the broader scope of our model reflects the inherent challenges of capturing all relevant factors in dynamic natural systems (Aguirre-Gutiérrez et al., 2013). The moderate R2 value in this study underscores these complexities while still providing meaningful insights into the influence of environmental drivers. The observed differences between our findings and those of other studies may result from regional climate variability and species-specific characteristics. South Korea’s distinct climate, including monsoons and harsh winters, introduces unique stressors that might not affect other bumblebee species studied in more temperate regions. Furthermore, B. h. sapporoensis may exhibit specific behavioral or physiological responses that differ from other well-studied species, influencing how it responds to climatic changes. Additionally, the moderate R2 suggests the possibility of unmeasured factors, such as pesticide exposure, habitat fragmentation, or floral diversity, which are known to affect pollinator populations but were not included in this study.
The model proves reliable and suitable for simulating the population dynamics of B. h. sapporoensis under climate change conditions. The validation metrics confirm that the model captures the critical environmental influences that drive population trends, despite the challenges inherent in ecological forecasting. The ability to integrate geographical variables such as elevation and latitude, alongside diverse climatic factors, makes this model a robust tool for assessing the future of bee populations under changing environmental conditions. These findings validate the model’s effectiveness in supporting ecological research and conservation planning, offering a strong foundation for future studies aimed at mitigating the impacts of climate change on B. h. sapporoensis.
2. Temporal analysis of climate change impacts on B. h. sapporoensis ecosystems
The study on the impacts of climate change on B. h. sapporoensis examines how climate factors, particularly rising temperatures, influence the distribution and activity of this bumblebee population (Kudo, 2013). Drawing from various studies, it is clear that climatic conditions-such as temperature, precipitation, and humidity-are critical in determining B. h. sapporoensis̓s activity and reproductive success, and that changes in these factors can significantly impact the survival and reproductive capacity of the population. South Korea̓s regions, like Gangwon and Jeju, offer a relatively favorable environment for B. h. sapporoensis during the summer due to cooler temperatures and higher humidity. Gangwon̓s mountainous climate exhibits significant temperature fluctuations but still supports favorable summer activity, aiding in the maintenance of local B. h. sapporoensis populations. In contrast, Jeollanam-do and Gyeongsangnam-do experience high temperatures and precipitation in summer, which can positively affect bumblebee activity, but they also have pronounced temperature variability and potential reductions in winter survival rates. In urban areas, the urban heat island effect can extend the activity period of B. h. sapporoensis due to relatively higher temperatures; however, habitat fragmentation and isolation may hinder the persistence of viable populations (Fig. 3).
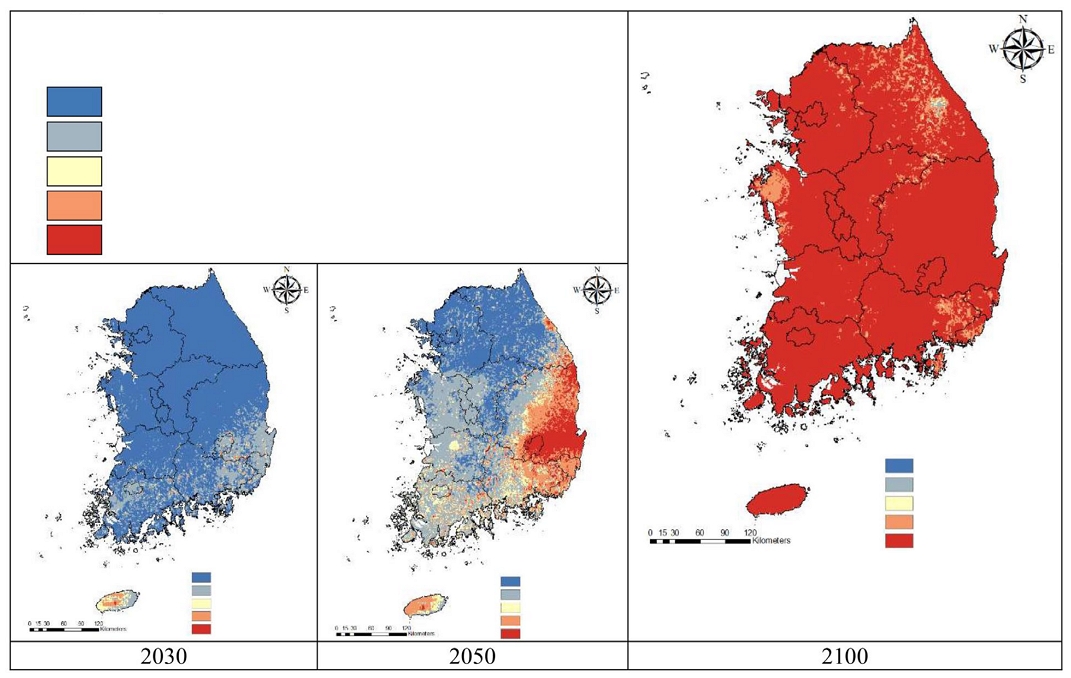
Future climate projected changes in average air temperature across South Korea for the years 2030, 2050, and 2100. These projections indicate a steady increase in temperature, with most regions reaching critical levels by 2100. Potentially limiting suitable habitat for B. h. sapporoensis.
According to climate change projections, rising summer temperatures and precipitation may narrow the suitable habitat range for B. h. sapporoensis. While summer activity could potentially increase, overall population decline may occur due to reduced winter survival rates. These predictions are crucial for evaluating B. h. sapporoensis̓s climate resilience and developing effective conservation strategies to mitigate population losses.
Temperature forecasts for 2030, 2050, and 2100 predict a significant rise in maximum temperatures across South Korea, with particularly pronounced warming in central and southern regions. Although Gangwon Province̓s higher elevation may maintain relatively cooler temperatures, extreme summer heat is likely to increase post-2050, potentially worsening habitat conditions. The Seoul metropolitan area is expected to experience marked temperature increases, with maximum temperatures projected to exceed 37.8°C by 2100, negatively impacting B. h. sapporoensis habitats. In Chungcheongnam-do and Chungcheongbuk-do, extended periods of high temperatures post-2050 could decrease habitat suitability, potentially pushing B. h. sapporoensis populations northward. Similarly, high-temperature stress is anticipated to reduce B. h. sapporoensis activity in Gyeongsangnam-do and Gyeongsangbuk-do, further challenging population stability. In Jeollanam-do and Jeollabuk-do, temperature rise and fluctuating precipitation patterns are expected to deteriorate habitat conditions, leading to population declines. Jeju is also likely to experience rapid temperature increases after 2050, diminishing its suitability as a habitat for B. h. sapporoensis (Fig. 4). By 2100, minimum temperatures across South Korea are projected to exceed 26.2°C, suggesting that B. h. sapporoensis will face increased thermal stress during both summer and winter. While Gangwon Province may retain relatively suitable conditions, the intensified summer and winter stress by 2100 could restrict the habitat range further northward. Similarly, temperature rises in metropolitan areas and the Chungcheong regions may create inhospitable conditions, while southern regions are expected to experience population declines due to thermal stress (Fig. 5).
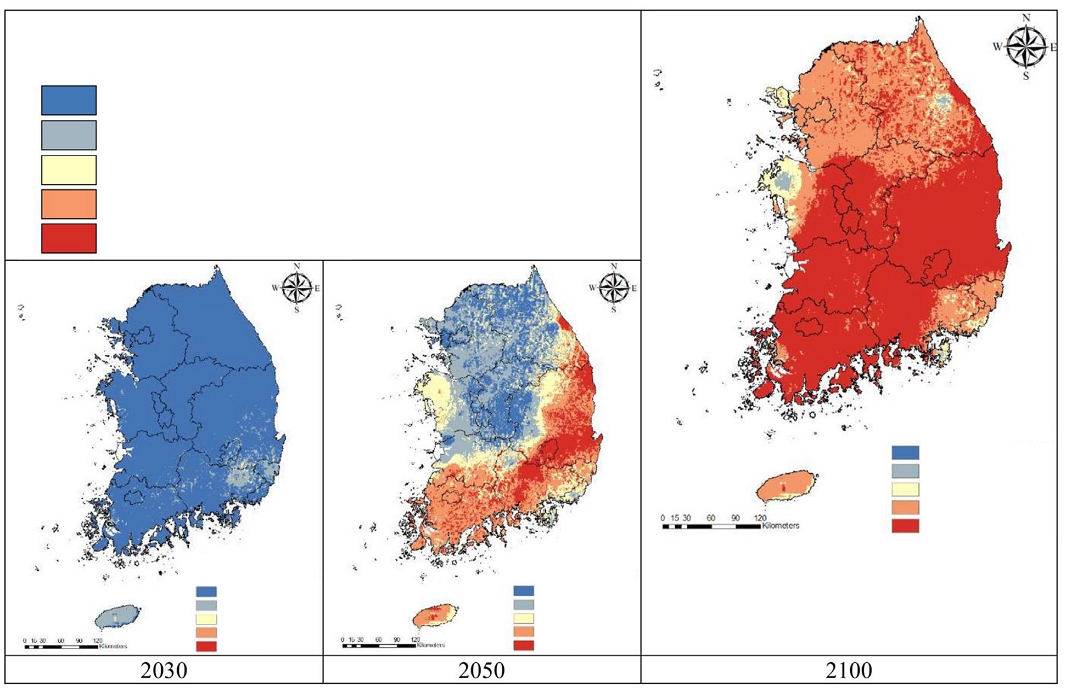
Future climate projected maximum air temperature changes in South Korea for 2030, 2050, and 2100. These projections reveal a substantial increase in maximum temperatures, especially in central and southern regions by 2100, potentially limiting suitable habitat for B. h. sapporoensis.
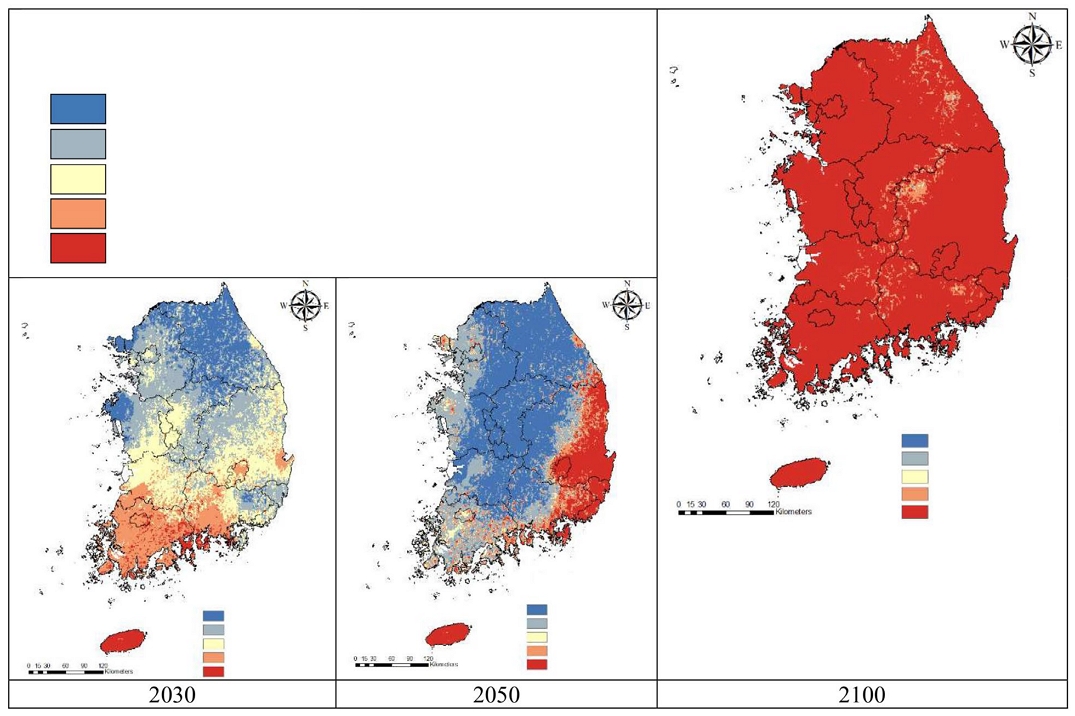
Future climate projected minimum air temperature changes in South Korea for 2030, 2050, and 2100, illustrating significant warming, with minimum temperatures expected to exceed 26.2°C across most regions by 2100. This increase in minimum temperatures could impose year-round thermal stress on B. h. sapporoensis.
The anticipated rise in average temperatures and reduction in diurnal temperature fluctuations are likely to adversely affect B. h. sapporoensis̓s ecology (Kim et al., 2021a). Increased average temperatures could limit breeding activities in spring and summer, and insufficient dormancy during hibernation could lower individual survival rates (Lenga and Huignard, 1992). Additionally, the reduced diurnal temperature variation may exacerbate thermal stress, impacting the physiological stability of B. h. sapporoensis (Block, 1981).
3. The impact of climate change on B. h. sapporoensis ecosystem resilience
The study examined nationwide temperature changes under climate change scenarios in relation to the activity of B. h. sapporoensis with a specific focus on the spring period and the hibernation preparation and hibernation period (Fig. 6).
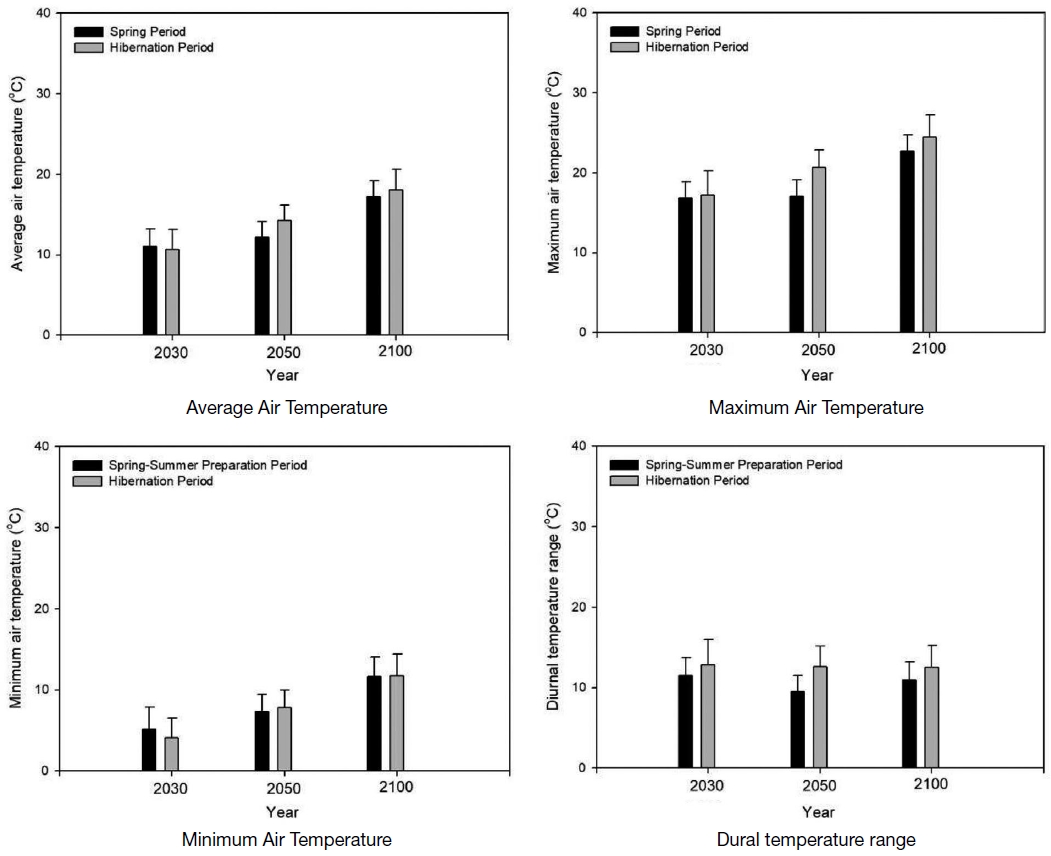
Future climate projected changes in average, maximum, minimum, and diurnal temperature ranges in South Korea for the spring-summer and hibernation periods in 2030, 2050, and 2100. Increasing temperatures and widening diurnal ranges suggest escalating thermal stress for B. h. sapporoensis, potentially impacting its seasonal behaviors and survival. Regional variability, as indicated by error bars, underscores the differing climate impacts across habitats.
According to these projections, both average and extreme temperatures across South Korea are expected to rise significantly by 2050 and 2100. Specifically, the average air temperature during the spring-summer period is projected to increase from around 25°C in 2030 to approximately 27°C by 2050 and nearly 30°C by 2100. Similarly, the average temperature during the hibernation period is expected to rise from around 15°C in 2030 to 17°C by 2050 and reach 20°C by 2100. The error bars representing nationwide temperature deviations indicate that the variability in average temperature across regions may range from ±2°C to ±3°C, highlighting considerable regional temperature differences, which may affect bee activity in varying ways across the country.
The projections indicate a marked increase in maximum air temperatures during the spring-summer period, rising from around 30°C in 2030 to 32°C by 2050 and exceeding 35°C by 2100. Similarly, maximum temperatures during the hibernation period are expected to climb from 20°C in 2030 to 23°C by 2050 and reach 25°C by 2100. The nationwide deviations in maximum temperatures are estimated to be approximately ±3°C, suggesting that while some regions may experience relatively moderate heat, others will endure more extreme maximum temperatures, potentially exacerbating local stress on B. h. sapporoensis populations, especially in warmer areas.
Minimum temperatures, which represent winter conditions, are also projected to rise. In the spring-summer period, minimum temperatures are expected to increase from around 20°C in 2030 to 22°C by 2050 and up to 25°C by 2100. During the hibernation period, minimums are anticipated to climb from around 5°C in 2030 to 7°C by 2050 and reach 10°C by 2100. The error bars for minimum temperatures indicate national deviations of approximately ±2°C, suggesting that certain regions may experience milder winter conditions while others may still face more extreme cold. This variability could result in uneven winter survival rates for the projections indicate a marked increase in maximum air temperatures during the spring-summer period, rising from around 30°C in 2030 to 32°C by 2050 and exceeding 35°C by 2100. Similarly, maximum temperatures during the hibernation period are expected to climb from 20°C in 2030 to 23°C by 2050 and reach 25°C by 2100. The nationwide deviations in maximum temperatures are estimated to be approximately ±3°C, suggesting that while some regions may experience relatively moderate heat, others will endure more extreme maximum temperatures, potentially exacerbating local stress on B. h. sapporoensis populations, especially in warmer areas.
The projected widening of the diurnal temperature range, which is the difference between daily maximum and minimum temperatures, represents increasing temperature variability across South Korea. This range is expected to stabilize around 12°C to 15°C by 2100, rising from about 10-12°C in 2030 to around 13°C by 2050, and reaching approximately 15°C by 2100. Nationwide deviations in the diurnal temperature range are anticipated to be around ±1°C to ±2°C, indicating that some regions will experience a larger temperature fluctuation compared to others. This variability could further challenge the adaptability of B. h. sapporoensis, as wider temperature swings are known to stress the physiological mechanisms of the bumblebees, particularly during the transition between active and hibernation periods.
This study suggests that climate change in South Korea could directly impact the breeding and survival of B. h. sapporoensis populations. Rising temperatures and decreasing diurnal temperature ranges may affect bumblebee activity, breeding cycles, and survival rates, potentially leading to population declines and habitat reduction (Naeem et al., 2019). Therefore, habitat protection and management measures to address climate change are necessary for the conservation of the Sapporo bumblebee population (Suzuki-Ohno et al., 2020).
4. B. h. sapporoensis population trends under climate change
The presented study visualizes the projected changes in Sapporo bumblebee population distribution across South Korea due to climate change, with forecasts for 2030, 2050, and 2100. The maps depict the expected population shifts using color gradients, where blue indicates low populations and red denotes high populations. This data helps us understand how future temperature changes may impact the B. h. sapporoensis. (Fig. 7).
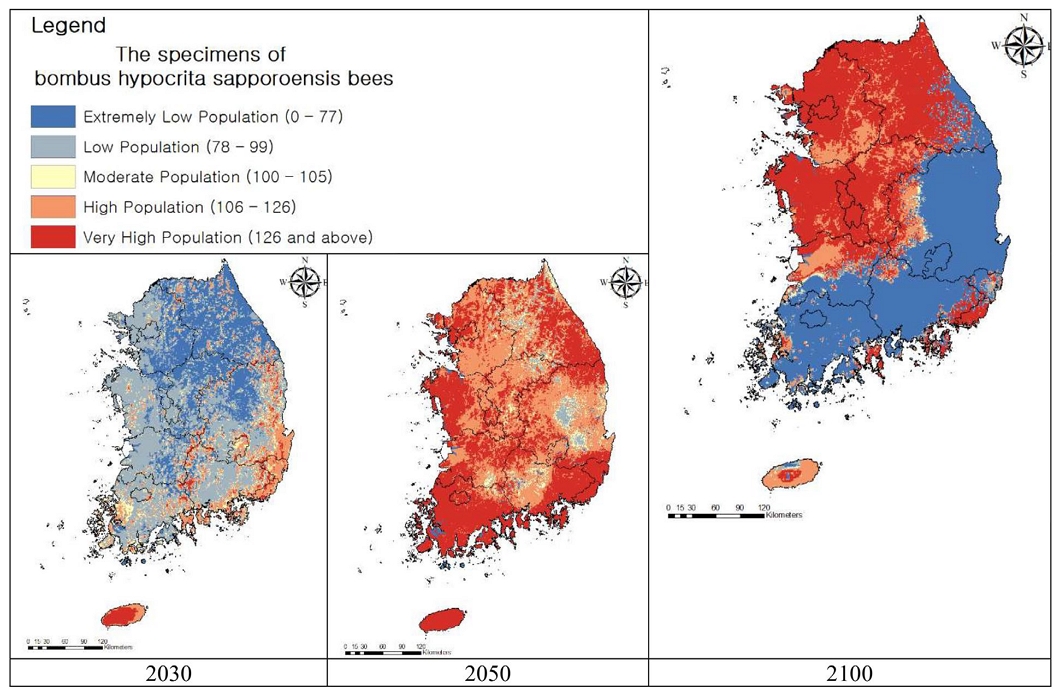
Future climate projections of B. h. sapporoensis populations in South Korea for 2030, 2050, and 2100. The maps illustrate a significant population increase in central and northern regions by 2100 due to climate change, with higher suitability for the species in these areas. Southern regions are projected to maintain lower populations, reflecting less favorable habitat conditions.
In 2030, most regions are projected to have B. h. sapporoensis populations in the “very low” or “low” range, with some southern and central areas showing “moderate” levels. By 2050, rising temperatures are expected to significantly boost populations in central and northern regions, reaching “high” and “very high” levels, while southern regions will still maintain low population levels. By 2100, the majority of regions across the country are projected to reach “very high” population levels, with the highest distribution increases observed in central and northern areas, and moderate or higher population levels anticipated in select southern and central regions. In Gangwon and northern Gyeonggi, populations in 2030 are predominantly in the “very low” or “low” range, indicating limited bumblebee habitation. By 2050, rising temperatures will lead to “moderate” to “high” population levels, particularly in northern Gangwon, and by 2100, population levels are expected to be “high” and “very high” across all of Gangwon, indicating increased habitat suitability. In Seoul, Gyeonggi, and Incheon (the capital region), populations in 2030 are expected to be in the “very low” or “low” range, with limited habitat suitability for the bumblebee. By 2050, populations will increase to “moderate” to “high” levels, indicating increased habitation in urban areas, and by 2100, most of the capital region is expected to reach “very high” levels, making it a viable habitat for the B. h. sapporoensis.
In Chungcheongnam-do and Chungcheongbuk-do, populations are expected to be in the “low” to “moderate” range in 2030, suggesting these regions have potential as habitats for the bumblebee. By 2050, populations are anticipated to increase rapidly, reaching the “high” range, and by 2100, most of the Chungcheong region is predicted to have “very high” population levels, establishing it as an important habitat for the species. In contrast, Jeollanam-do and Jeollabuk-do, the southern regions, are expected to be predominantly in the “very low” to “low” range in 2030, indicating unsuitable habitat conditions. While some increase in populations is expected in southern areas by 2050, habitat conditions will remain limited. By 2100, only some areas may reach “moderate” population levels, and the region̓s role as a habitat will likely remain limited. Similarly, in Gyeongsangnam-do and Gyeongsangbuk-do, populations are expected to be in the “very low” to “low” range in 2030, suggesting unsuitable conditions for habitation. By 2050, northern Gyeongsangbuk-do may see an increase to “moderate” levels, but overall low populations are anticipated. By 2100, due to rising temperatures, the suitability of these regions as habitats will likely remain low. This study indicates that the B. h. sapporoensis population in South Korea may increase significantly in certain regions due to climate change. Central and northern regions, in particular, are likely to become more suitable habitats, which is closely related to habitat shifts driven by rising temperatures.
The findings of this study highlight the complex and dynamic relationship between climate change and species distribution. The projected increase in the B. h. sapporoensis population in central and northern regions suggests that rising temperatures may create more favorable conditions for this species in areas that were previously less suitable. This shift could be attributed to a combination of factors, including increased availability of forage plants, suitable nesting sites, and temperature ranges that better support bumblebee activity and reproduction (Suzuki-Ohno et al., 2020). However, these changes also raise concerns about the long-term stability of B. h. sapporoenisis, particularly in southern regions where rising temperatures may create conditions that are too extreme for the Sapporo bumblebee to thrive. The decrease in suitable habitats in southern areas underscores the importance of monitoring these populations and implementing conservation strategies to mitigate the adverse effects of climate change. The loss of bumblebee populations in southern regions could have significant ecological consequences, as bumblebees are important pollinators that contribute to the health and diversity of ecosystems (Cameron et al., 2011).
SUMMARY
This study provides significant insights into how the ecology and population dynamics of Bombus hypocrita sapporoensis are influenced by climate change, offering important implications for its long-term survival. The research indicates that under climate change scenarios, particularly the SSP 5.85 high-emission scenario, B. h. sapporoensis is likely to shift its habitat northward as rising temperatures render current habitats less suitable. The analysis of climate indices reveals that specific weather conditions significantly impact the population size of this species. For instance, optimal spring weather conditions positively influence population size, while excessively hot or cold spring days reduce it. Additionally, high temperatures and precipitation negatively affect bee activity and resource accumulation, thereby lowering survival rates.
In areas with higher elevations, B. h. sapporoensis populations tend to thrive due to cooler climates, fewer human disturbances, and reduced competition from other species. These high-elevation regions provide a more favorable environment for the bumblebees, allowing them to establish stable populations. Likewise, populations in higher latitudes also exhibit a slight increase in size, as the cooler temperatures and longer seasons in northern regions better suit the species̓ ecological requirements. The regression model developed in this study explains 91.8% of the variance in population size, underscoring the importance of geographic and environmental factors such as latitude, elevation, and seasonal weather patterns in shaping population dynamics. These geographic and climatic factors play a crucial role in determining the suitability of habitats for B. h. sapporoensis, and understanding their influence is essential for developing effective conservation strategies for this important pollinator species.
Despite these valuable findings, the study has some limitations. First, the reliance on the SSP 5.85 high-emission scenario assumes an extreme climate future, which may not fully reflect actual future conditions if mitigation efforts are successful. Additionally, while the regression model demonstrates high explanatory power, it does not comprehensively account for other critical factors such as pesticide exposure, habitat fragmentation, or floral diversity-elements that could also significantly influence population dynamics. Furthermore, the study emphasizes the importance of geographical factors, it does not thoroughly explore how human activities or competition with other species might affect B. h. sapporoensis populations. Consequently, future research should incorporate various climate change scenarios, as well as the impacts of human activities and interspecific competition, to develop more comprehensive conservation strategies for this important pollinator species.
This study offers valuable insights into the impact of climate change on the ecology and population dynamics of B. h. sapporoensis, providing crucial data to inform strategies addressing future changes. Despite some limitations, the findings can significantly contribute to the development of conservation strategies for this important pollinator species.
Acknowledgments
This research was supported by the Rural Development Administration research project (PJ0158702).
References
-
Aguirre-Gutiérrez, J., L. G. Carvalheiro, C. Polce, E. E. V. Loon, N. Raes, M. Reemer and J. C. Biesmeijer. 2013. Fit-for-purpose: species distribution model performance depends on evaluation criteria - Dutch hoverflies as a case study. PLoS One 8(5): e63708.
[https://doi.org/10.1371/journal.pone.0063708]
-
An, S., G. Park, H. Jung and D. Jang. 2022. Assessment of future drought index using SSP scenario in Rep. of Korea. Sustainability 14(7): 4252-4252.
[https://doi.org/10.3390/su14074252]
-
Banks, H. T., J. E. Banks, R. Bommarco, A. N. Laubmeier, N. Myers, M. Rundlöf and K. Tillman. 2017. Modeling bumble bee population dynamics with delay differential equations. Ecol. Model. 351: 14-23.
[https://doi.org/10.1016/j.ecolmodel.2017.02.011]
-
Barbieri, F., F. Bernini, F. M. Guarino and A. Venchi. 2010. Distribution and conservation status of Bombina variegata in Italy. Ital. J. Zool. 6: 83-90.
[https://doi.org/10.1080/11250003.2004.9525565]
-
Block, W. 1981. Terrestrial arthropods and low temperature. Cryobiology 18(4): 436-444.
[https://doi.org/10.1016/0011-2240(81)90119-X]
-
Cameron, S. A., J. D. Lozier, J. P. Strange, J. B. Koch, N. Cordes, L. F. Solter and T. Griswold. 2011. Patterns of widespread decline in North American bumble bees. Proc. Natl. Acad. Sci. USA 108(2): 662-667.
[https://doi.org/10.1073/pnas.1014743108]
-
Change, I. P. O. C. 2023. Annex VI: climatic impact-driver and extreme. Indices 2205-2214.
[https://doi.org/10.1017/9781009157896.020]
-
Clarke, D. and D. Robert. 2018. Predictive modelling of honey bee foraging activity using local weather conditions. Apidologie 49(3): 386-396.
[https://doi.org/10.1007/s13592-018-0565-3]
-
Dolan, A. C., C. M. Delphia, K. M. O’neill and M. A. Ivie. 2016. Bumble bees (Hymenoptera: Apidae) of Montana. Ann. Entomol. Soc. Am. 110(2): 129-144.
[https://doi.org/10.1093/aesa/saw064]
-
Dunne, J. P., J. G. John, E. Shevliakova, R. J. Stouffer, J. P. Krasting, S. Malyshev, P. C. D. Milly, L. T. Sentman, A. Adcroft, W. Cooke, K. A. Dunne, S. M. Griffies, R. Hallberg, M. Harrison, H. Levy, A. T. Wittenberg, P. J. Phillips and N. Zadeh. 2012. GFDL’s ESM2 global coupled climate-Carbon earth system models. Part II: Carbon system formulation and baseline simulation characteristics. J. Clim. 26(7): 2247-2267.
[https://doi.org/10.1175/JCLI-D-12-00150.1]
-
Ghassemi-Khademi, T., R. Khosravi, S. Sadeghi and M. Ebrahimi. 2021. Historical, current, and future climate niche of the red dwarf honey bee across its native range. J. Apic. Res. 61(2): 271-283.
[https://doi.org/10.1080/00218839.2021.1892370]
-
Jaboor, S. K., C. R. B. D. Silva and V. Kellermann. 2022. The effect of environmental temperature on bee activity at strawberry farms. Austral Ecol. 47(7): 1470-1479.
[https://doi.org/10.1111/aec.13228]
-
Jang, K., M. Won and S. Yoon. 2017. Evaluation of the satellite-based air temperature for all sky conditions using the automated mountain meteorology station (AMOS) records: Gangwon Province case study. Korean J. Agric. For. Meteorol. 19(1): 19-26.
[https://doi.org/10.5532/KJAFM.2017.19.1.19]
-
Karbassioon, A., J. Yearlsey, T. Dirilgen, S. Hodge, J. C. Stout and D. A. Stanley. 2023. Responses in honeybee and bumblebee activity to changes in weather conditions. Oecologia 201(3): 689-701.
[https://doi.org/10.1007/s00442-023-05332-x]
-
Kim, H. W., P. Adhikari, M. H. Chang and C. Seo. 2021a. Potential distribution of amphibians with different habitat characteristics in response to climate change in South Korea. Animals 11(8): 2185-2185.
[https://doi.org/10.3390/ani11082185]
-
Kim, J. P. 2014. Estimation of high resolution daily precipitation using a modified PRISM model. KSCE J. Civ. Eng. 34(4): 1139-1139.
[https://doi.org/10.12652/Ksce.2014.34.4.1139]
- Kim, S., T. K. Kim, S. Yoon, K. Jang, H. Lim, W. Y. Lee, M. Won, J. Lim and H. S. Kim. 2021b. Recent changes in bloom dates of Robinia pseudoacacia and bloom date predictions using a process-based model in South Korea. J. Korean Soc. For. Sci. 110(3): 322-340.
-
Kudo, G. 2013. Vulnerability of phenological synchrony between plants and pollinators in an alpine ecosystem. Ecol. Res. 29(4): 571-581.
[https://doi.org/10.1007/s11284-013-1108-z]
-
Kwon, Y. J. and S. Saeed. 2003. Effect of temperature on the foraging activity of Bombus terrestris L. (Hymenoptera: Apidae) on greenhouse hot pepper (Capsicum annuum L). Appl. Entomol. Zool. 38(3): 275-280.
[https://doi.org/10.1303/aez.2003.275]
-
Kwon, Y. J. 2008. Bombiculture: a fascinating insect industry for crop pollination in Korea. Entomol. Res. 38(1): 66-70.
[https://doi.org/10.1111/j.1748-5967.2008.00176.x]
-
Lenga, A. and J. Huignard. 1992. Effect of changes in the thermoperiod on reproductive diapause in Bruchidius atrolineatus Pic (Coleoptera: Bruchidae). Physiol. Entomol. 17(3): 247-254.
[https://doi.org/10.1111/j.1365-3032.1992.tb01018.x]
-
Naeem, M., M. Liu, J. Huang, G. Ding, G.S. Potapov, C. Jung and J. An. 2019. Vulnerability of East Asian bumblebee species to future climate and land cover changes. Agric. Ecosyst. Environ. 277: 11-20.
[https://doi.org/10.1016/j.agee.2019.03.002]
- Nagamitsu, T., M. Kato and K. Inoue. 2005. Pollen utilization by three colonies of B. h. sapporoensis colonies in fragmented forests landscapes in Hokkaido, Japan. Bulletin of FFPRI. 395: 167-175.
-
Papanikolaou, A. D., I. Kuhn, M. Frenzel and O. Schweiger. 2017. Semi-natural habitats mitigate the effects of temperature rise on wild bees. J. Appl. Ecol. 54(2): 527-536.
[https://doi.org/10.1111/1365-2664.12763]
-
Potts, S. G., J. C. Biesmeijer, C. Kremen, P. Neumann, O. Schweiger and W. E. Kunin. 2010. Global pollinator declines: trends, impacts and drivers. Trends Ecol. Evol. 25(6): 345-353.
[https://doi.org/10.1016/j.tree.2010.01.007]
-
Rahimi, E. and C. Jung. 2024. Global trends in climate suitability of bees: Ups and downs in a warming world. Insects 15(2): 127.
[https://doi.org/10.3390/insects15020127]
-
Rahimi, E., S. Barghjelveh and P. N. Dong. 2021. Estimating potential range shift of some wild bees in response to climate change scenarios in northwestern regions of Iran. J Ecol. Environ. 45(14): 1-13.
[https://doi.org/10.1186/s41610-021-00189-8]
-
Rao, S., Z. Klimont, S. J. Smith, R. V. Dingenen, F. Dentener, A. F. Bouwman, K. Riahi, M. Amann, B. L. Bodirsky, D. P. Vuuren, L. A. Reis, K. Calvin, L. Drouet, O. Fricko, S. Fujimori, D. Gernaat, P. Havlík, M. Harmsen, T. Hasegawa and M. Tavoni. 2016. Future air pollution in the Shared Socio-economic Pathways. Glob. Environ. Change 42: 346-358.
[https://doi.org/10.1016/j.gloenvcha.2016.05.012]
-
Sirois-Delisle, C. and J. T. Kerr. 2018. Climate change-driven range losses among bumblebee species are poised to accelerate. Sci. Rep. 8(1): 14464.
[https://doi.org/10.1038/s41598-018-32665-y]
-
Suzuki-Ohno, Y., J. Yokoyama, T. Nakashizuka and T. M. Kawata. 2020. Estimating possible bumblebee range shifts in response to climate and land cover changes. Sci. Rep. 10(1): 19622.
[https://doi.org/10.1038/s41598-020-76164-5]
-
Takeuchi, T., M. Takahashi, M. Nishimoto, T. Kiyoshi, K. Tsuchida, T. Nomura and J. Takahashi. 2018. Genetic structure of the bumble bee Bombus hypocrita sapporoensis. a potential domestic pollinator for crops in Japan. J. Apic. Res. 57(2): 203-212.
[https://doi.org/10.1080/00218839.2017.1412879]
-
Tennakoon, S., A. Apan and T. Maraseni. 2024. Unravelling the impact of climate change on honey bees: An ensemble modelling approach to predict shifts in habitat suitability in Queensland, Australia. Ecol. Evol. 14(4): 1-19.
[https://doi.org/10.1002/ece3.11300]
-
Tsuchida, K., A. Yamaguchi, Y. Kanbe and K. Goka. 2019. Reproductive interference in an introduced bumblebee: Polyandry may mitigate negative reproductive impact. Insects 10(2): 59.
[https://doi.org/10.3390/insects10020059]
-
Weiskopf, S. R., M. A. Rubenstein, L. G. Crozier, S. Gaichas, R. B. Griffis, J. E. Halofsky, K. J. Hyde, T. L. Morelli, J. T. Morisette, R. C. Muñoz, A. J. Pershing, D. L. Peterson, R. Poudel, M. D. Staudinger, A. E. Sutton-Grier, L. M. Thompson, J. M. Vose, J. F. Weltzin and K. P. Whyte. 2020. Climate change effects on biodiversity, ecosystems, ecosystem services, and natural resource management in the United States. Sci. Total Environ. 733(1): 137782.
[https://doi.org/10.1016/j.scitotenv.2020.137782]
-
Wilson, J. D., O. Andrews, A. Katavouta, F. D. M. Viríssimo, R. Death, M. Adloff, C. Baker, B. Blackledge, F. W. Goldsworth, A. Kennedy-Asser, Q. Liu, K. Sieradzan, E. Vosper and R. Ying. 2022. The biological carbon pump in CMIP6 models: 21st century trends and uncertainties. Proc. Natl. Acad. Sci. USA 119(29): e2204369119.
[https://doi.org/10.1073/pnas.2204369119]
-
Yokoyama, J. and M. N. Inoue. 2010. Status of the invasion and range expansion of an introduced bumblebee, Bombus terrestris (L.), in Japan. Appl. Entomol. Zool. 45(1): 21-27.
[https://doi.org/10.1303/aez.2010.21]
-
Yoon, H. J., S. E. Kim and Y. S. Kim. 2002. Temperature and humidity favorable for colony development of the indoor-reared bumblebee, Bombus ignitus. Appl. Entomol. Zool. 37(3): 419-423.
[https://doi.org/10.1303/aez.2002.419]