
Simulating Honeybee (Apis mellifera) Winter Mortality Rate under Climate Change Scenarios: Development and Application of Meteorological Index-Based Predictive Models
Abstract
This study investigates the role of winter meteorological indices in predicting Apis mellifera colony losses under various climate change scenarios. This predictive model focuses on environmental temperature variability, emphasizing extreme fluctuations and persistent cold spells, which significantly contribute to overwintering mortality. The findings indicate that rising average temperatures and an increase in high-temperature days have the most profound impact on A. mellifera overwintering mortality. Additionally, an expanded daily temperature range, especially in southern and inland regions, further complicates the bees' ability to regulate their temperature, leading to additional energy expenditures that weaken colony resilience. Furthermore, the projected decrease in low-temperature days by 2100, particularly in southern coastal and central inland areas, presents a challenge for bees, as reduced exposure to cold disrupts their ability to transition into the overwintering phase, leaving colonies unprepared for winter. Moreover, the increase in autumn rainfall days threatens bees by limiting their foraging days and hindering their ability to stockpile resources for winter. This study developed meteorological index-based predictive models to simulate A. mellifera winter mortality rate across the country and applied these models to evaluate the potential impacts of future climate change scenarios on A. mellifera overwintering mortality. The findings emphasize the need for region-specific weather-based forecasting models, as climate impacts on A. mellifera survival vary across different geographic areas. The development of such predictive tools can inform strategic management decisions to mitigate the effects of climate change on pollinator populations and maintain the critical ecosystem services.
Keywords:
Apis mellifera, Overwintering mortality, Climate change, Meteorological index, Predictive modelINTRODUCTION
Apis mellifera colony collapse has emerged as a significant global crisis, with growing concerns about the impact of climate change on A. mellifera survival (van Engelsdorp et al., 2008). A. mellifera play a vital role in ecosystems, contributing to plant pollination and food production (Calovi et al., 2021; Rajagopalan et al., 2024). In South Korea, the problem of A. mellifera winter mortality has become increasingly severe in recent years, with factors such as abnormal temperatures, pests, and nutritional deficiencies exacerbating this concerning trend (Kim et al., 2016; Truong et al., 2023). Climate change is a major factor influencing the success of A. mellifera overwintering. Several studies have identified the mechanisms by which climate change leads to increased A. mellifera mortality (Quinlan et al., 2023; Rajagopalan et al., 2024). The temperature variability associated with climate change directly impacts the survival of A. mellifera colonies, weakening their resilience and making them more susceptible to pests and diseases (Switanek et al., 2016; Calovi et al., 2021). These temperature fluctuations can stress the bees, causing them to expend more energy to maintain a stable hive temperature, which in turn can reduce their overall fitness and make them more vulnerable to pathogens and parasites (Calovi et al., 2021). Additionally, warmer temperatures and erratic weather patterns can create favorable conditions for the proliferation of pests, such as Varroa mites (Varroa destructor) and Nosema, leading to increased infestations and elevated colony mortality rates (Overturf et al., 2022). Lastly, dramatic temperature fluctuations, particularly wide daily temperature ranges, can disrupt the A. mellifera ability to maintain a stable hive temperature, further compromising their survival (Desai and Currie, 2016).
The key meteorological factors that influence A. mellifera overwintering mortality are as follows: extreme temperature fluctuations, particularly rapid temperature drops in winter, which are a critical factor contributing to the decline in A. mellifera colony survival rates (Mardan and Kevan, 2002). A. mellifera expend significant energy to maintain a consistent hive temperature, and substantial temperature variations can increase their energy expenditure, weaken them, and make them more susceptible to diseases (Heinrich, 1996; Harrison and Fewell, 2002). Similarly, large daily temperature differences can negatively impact A. mellifera survival (Stabentheiner and Kovac, 2014). Excessive precipitation or heavy snowfall during the winter can also interfere with the foraging activities of A. mellifera and increase humidity, which elevates the risk of disease outbreaks (Abou-Shaara et al., 2017). Reduced sunlight exposure during cloudy or overcast weather conditions can also negatively impact the bees’ ability to maintain their internal hive temperature (Li et al., 2019). These meteorological factors do not act in isolation, but rather exert a combined influence on A. mellifera overwintering mortality. To enhance the survival rate of A. mellifera, a comprehensive analysis and development of predictive models that consider various meteorological elements is necessary to better understand and mitigate the challenges posed by climate change on A. mellifera populations.
To address the growing importance of understanding the interactions between climate change and A. mellifera overwintering mortality, it is critical to simulate the potential impacts of future climate scenarios on A. mellifera populations. By simulating different climate change scenarios, it is possible to predict how changes in temperature, precipitation, and other weather factors will influence A. mellifera colony health and overwintering success (Switanek et al., 2016). This approach will allow researchers and stakeholders to anticipate potential risks and develop adaptive management strategies to protect A. mellifera populations. While some studies have focused on the influence of specific meteorological variables or management practices, there remains a lack of comprehensive research on the broader, complex interactions between climate change and A. mellifera colony collapse in South Korea.
This research aims to develop a comprehensive set of meteorological indices that capture the optimal environmental conditions for A. mellifera activity and survival. These indices will synthesize key factors, such as temperature, precipitation, and daily temperature range, that are known to significantly impact A. mellifera health and colony viability (Becsi et al., 2021). The introduction of these meteorological indices offers several advantages: enhanced accuracy of predictive models, a holistic analysis of the complex relationships between climate change and A. mellifera mortality, and improved ability to anticipate and respond to the challenges posed by climate change (Calovi et al., 2021).
The primary research objectives of this study are threefold: 1) to develop a set of four distinct meteorological indices that comprehensively capture the optimal environmental conditions required for A. mellifera activity and survival, 2) to construct predictive models for A. mellifera overwintering mortality based on these newly developed meteorological indices, and 3) to apply these models to climate change scenarios to evaluate the potential impacts on A. mellifera winter mortality rate under future climate conditions. By expanding our understanding of the intricate connections between meteorological factors and A. mellifera survival, this research aims to provide valuable insights and tools to support the protection and resilience of A. mellifera populations in the face of a changing climate.
METHODOLOGY
1. Field survey on Apis mellifera winter mortality rates
The study gathered data from 2022 to 2023 by surveying 200 beekeeping farms across the country in 2022 and 40 farms in 2023. A combination of structured surveys and on-site visits was used to collect information about the practices, challenges, and outcomes associated with Apis mellifera colony survival over the winter. The data collected included variables such as geographic location, beekeeper experience, migratory practices, colony counts before and after wintering, and winter mortality rates. The 200 farms surveyed in 2022 were used to build the predictive models, while the 40 farms surveyed in 2023 were used to validate the models.
The winter mortality rate was calculated by comparing the number of A. mellifera colonies in October before overwintering to the number of colonies remaining in March after overwintering. This measurement represents the percentage of colonies that did not survive the winter period, providing a crucial indicator for evaluating the impact of environmental, biological, and management factors on bee populations during the particularly vulnerable winter season. Mathematically, the winter mortality rate can be expressed as:
This measurement is significant in evaluating the impact of environmental, biological, and management factors on bee populations during winter-a period when resources are scarce and colonies are particularly vulnerable (Kulhanek et al., 2017).
High winter mortality rates typically suggest that A. mellifera colonies face stressors such as inadequate food stores, harsh climate conditions, disease, or suboptimal management practices (Steinhauer et al., 2014). The geographic information of each farm, including latitude and longitude, was used to generate spatial information for the beekeeping farms in 2022 and 2023. The 200 farms surveyed in 2022 were used to build the raster, while 35 farms surveyed in 2023 were used for raster validation. Fig. 1 shows the locations of the beekeeping farms surveyed in 2022 and 2023, which were mapped using ArcGIS 10.5 (ESRI, Redlands, CA, USA) software.
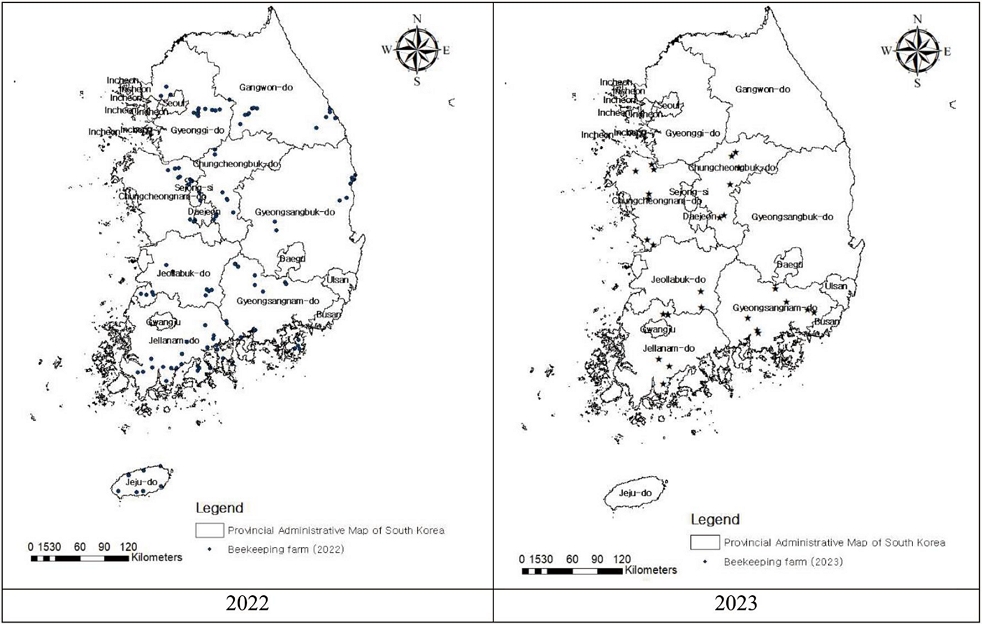
Modeling and validation of Apis mellifera winter survival: insights from nationwide beekeeping surveys (2022-2023).The study collected data from 2022 to 2023 by surveying 200 beekeeping farms 2022 to develop predictive models and 40 farms in 2023 for validation. Information on the winter survival of A. mellifera colonies, including variables such as geographic location, beekeeper experience, migratory practices, colony counts, and mortality rates, was obtained through structured surveys and on-site visits
2. Meteorological data collection and processing
The meteorological data used in this study were obtained from multiple reliable institutions, creating a comprehensive dataset that captured both general and locationspecific weather conditions. Observational data primarily came from the Korean Meteorological Administration, utilizing their Automatic Surface Observing System (ASOS) with 95 stations and Automatic Weather Stations (AWS) with 520 stations. The Korea Forest Service also contributed data from 464 Automatic Mountain Meteorology Observation Stations (AMOS), which monitor weather conditions at elevations exceeding 200 meters (Jang et al., 2017). This integration of data sources ensured comprehensive coverage of the diverse climatic conditions across Korea’s varied terrain, including both lowland and mountainous regions (Park et al., 2018). The dataset included daily records of maximum, minimum, and average temperatures, as well as precipitation. The observational datasets underwent rigorous preprocessing, including handling missing values, normalization, and scaling of variables to ensure consistency and data quality for further analysis. The diurnal temperature range, calculated as the difference between the daily maximum and minimum temperatures, was a crucial meteorological variable for analyzing the environmental impacts on Apis mellifera overwintering mortality. Several studies have examined the relationship between diurnal temperature range and A. mellifera overwintering mortality. These studies have found that significant variations in daily temperatures can negatively impact A. mellifera survival and colony health. Rapid temperature changes, especially sudden temperature drops, can disrupt the bees’ ability to maintain the optimal hive environment needed for successful overwintering, leading to increased energy expenditure and depletion of essential winter food stores.
The inclusion of data from both the Korean Meteorological Administration and the Korea Forest Service provided a comprehensive and accurate representation of the various climatic conditions across different regions and elevations in South Korea, facilitating a thorough investigation of the environmental factors influencing A. mellifera overwintering mortality.
3. Climate scenario projections
The study used climate projections from the GFDL-ESM2G Earth System Model, a comprehensive modeling framework. This model has a robust carbon cycle formulation that can capture the complex interactions between climate and carbon cycling under human influence. The GFDL-ESM2G model simulates dynamic ocean processes and interactive carbon dynamics, providing a more accurate representation of climate responses, particularly regarding ocean ventilation and nutrient cycling (Dunne et al., 2013). These oceanographic processes significantly impact carbon sequestration and the biological carbon pump. Additionally, the model incorporates dynamic vegetation, enabling a holistic representation of Earth system processes and their interdependencies (Siegel et al., 2022).
In this study, the high-emission SSP 5.85 scenario, which represents a future with limited climate change mitigation efforts, was utilized (An et al., 2022). These SSP scenarios provided projections of daily maximum, minimum, and average temperatures, as well as daily precipitation data. Diurnal temperature ranges were calculated by subtracting daily minimum temperatures from daily maximum temperatures. The modeled climate data served as the foundation for assessing the potential impacts of future climate change on A. mellifera overwintering mortality.
This study employed the high-emission SSP 5.85 scenario from the GFDL-ESM2G Earth System Model, which represents a future with limited climate change mitigation efforts, to simulate the potential impacts of climate change on A. mellifera overwintering mortality. This comprehensive climate modeling approach, combined with the detailed meteorological data, enabled a thorough investigation of the complex environmental factors influencing A. mellifera overwintering mortality in South Korea (Lim et al., 2018). The daily weather scenario data were collected from the Climate Information Portal (http://www.climate.go.kr) provided by the Korea Meteorological Administration.
4. Spatial interpolation and climate raster generation
To generate continuous spatial data across the study area, two interpolation techniques were employed: Inverse Distance Weighting for temperature and MK-PRISM for precipitation. The choice of each method was based on the specific characteristics of the climate variable being modeled, considering the topographical and environmental factors.
IDW was used to estimate temperature values, where unknown values were calculated as a weighted average of nearby known data points, with closer points having greater influence (Özelkan et al., 2013; Yan et al., 2015). However, IDW does not inherently account for the significant temperature lapse rate effects in mountainous regions, which can lead to biased estimates, particularly at higher elevations (Kim, 2014). To address this, adjustments for temperature lapse rates were applied, as previous research has demonstrated that temperature variability in mountainous areas is strongly influenced by elevation, necessitating the consideration of lapse rates to accurately reflect temperature gradients (Minder et al., 2010). This approach is particularly effective when the meteorological station network is dense, ensuring that local temperature variations are well represented.
The MK-PRISM approach was employed for precipitation modeling. Precipitation is influenced by more than just the distance from meteorological stations, unlike temperature. To capture the complex spatial patterns of precipitation across the study region, particularly the nuanced effects of mountainous terrain, the MK-PRISM regression approach was employed. This approach accounts for topographical characteristics, such as elevation, slope, and aspect, which have significant effects on precipitation distribution (Daly et al., 2008; Kim, 2014). By incorporating these terrain characteristics, high-resolution climate rasters were generated that effectively capture the variability in precipitation influenced by Korea’s diverse topography.
5. Climate indices for overwintering Apis mellifera
The overwintering success of Apis mellifera colonies is largely determined by specific meteorological conditions, which significantly impact bee health, foraging activities, and overall survival rates (Calovi et al., 2021). To better assess the climate’s influence on overwintering A. mellifera, a series of climate indices were developed incorporating temperature, precipitation, and diurnal temperature range. These indices are crucial for understanding how environmental conditions shape A. mellifera behavior and colony strength, especially during critical seasonal transitions (Becsi et al., 2021).
The Spring to Autumn Optimal Climate Index represents the ideal conditions for A. mellifera activity from March to November. During this period, a higher average temperature encourages efficient foraging, while limited precipitation and stable diurnal temperatures minimize stress. By maintaining favorable environmental conditions, colonies can maximize resource accumulation and maintain strong populations leading into winter.
Conversely, the Autumn Low Temperature Index indicates the early arrival of cold temperatures, which restricts foraging and forces bees to expend more energy maintaining the optimal hive temperature. When temperatures average below 10℃, with minimums dipping below 4℃, bees reduce their activity, increasing the risk of colony mortality due to insufficient food stores and energy demands (Owen et al., 2013; McAfee et al., 2020). On the other hand, warmer autumn conditions can indeed be detrimental to A. mellifera colonies, as they disrupt the bees’ natural overwintering processes. Higher autumn temperatures, with an average of at least 14℃ and minimums of at least 4℃, may extend the resource collection period, but can also prevent the colonies from adequately preparing for the harsh winter conditions (Rajagopalan et al., 2024). This can lead to several adverse effects, including prolonged foraging and energy expenditure, disruption of overwintering preparation such as forming winter clusters and conserving energy, skewed colony age structure resulting in “spring dwindle,” and increased pest pressure from pests like Varroa mites (Sgolastra et al., 2011). In contrast, lower autumn temperatures are more favorable for A. mellifera, as they allow the colonies to better transition into and prepare for the winter season (Ricigliano et al., 2018).
Precipitation also plays a key role in colony success (Switanek et al., 2016). The Autumn Precipitation Index measures cumulative rainfall above 0.5 mm from September to November, which can negatively impact A. mellifera foraging. Increased rainfall limits bee movement and resource collection, increasing colony stress. During winter, precipitation and temperature changes can further strain bee colonies, especially when paired with fluctuating diurnal temperatures (Switanek et al., 2016; Calovi et al., 2021).
The Winter Low Temperature Index is crucial for assessing harsh winter conditions. It considers periods of sustained low temperatures following high winter temperatures. A minimum temperature below 4℃ and a diurnal temperature range greater than or equal to 14℃ pose challenges for bee colonies. High variations in daily temperatures place additional stress on the bees, as they must constantly adjust to fluctuating conditions to maintain an optimal internal hive environment. This index is vital for understanding the energy expenditure and nutritional depletion experienced by overwintering colonies (Schenk et al., 2018; Maes et al., 2021). This index is vital for understanding the energy expenditure required for hive thermoregulation during the coldest months. In contrast, the Winter High-Temperature Index identifies periods when the average temperature exceeds 4℃, with minimum temperatures at or above 14℃. These warmer conditions can be both beneficial and harmful. While reduced energy requirements for maintaining warmth are advantageous, the increased activity due to higher temperatures may lead to greater resource consumption. This situation can leave colonies vulnerable if a subsequent cold spell occurs and resources are depleted prematurely (Sinclair, 2014).
Overall, these climate indices provide an essential framework for evaluating the overwintering success of A. mellifera colonies. Each index reflects critical aspects of the climate that can either benefit or harm bee populations (Becsi et al., 2021). By analyzing these indices, researchers and beekeepers can identify environmental stressors and develop mitigation strategies to enhance colony survival through challenging winter periods (Table 1).
6. Statistical modeling of overwintering mortality of Apis mellifera
To evaluate the relationship between seasonal weather measurements and Apis mellifera overwintering survival, a multiple linear regression model with backward elimination was utilized. The independent variables in the model included various seasonal weather measurements, while the dependent variable was the overwintering mortality rate of A. mellifera colonies. Preprocessing of the data was performed to enhance the quality and reliability of the analysis. This involved handling missing data through either imputation or removal, depending on the extent and potential impact of the missing values. Furthermore, normalization and scaling were applied to ensure that all variables were on a comparable scale, thereby reducing biases in the regression model. Correlation analysis was conducted to identify significant relationships between the weather measurements and overwintering mortality, highlighting the key climatic factors affecting A. mellifera survival. The regression model was constructed using data collected in 2022 and validated with data from 2023, ensuring that the model’s predictions were based on recent real-world observations. This methodology enabled the quantification of the effects of various climatic factors on the overwintering success and survival of A. mellifera colonies, thus providing a foundation for making accurate projections regarding population dynamics under future climate scenarios. The performance of the model was assessed using the coefficient of determination, R2, which offered insights into its explanatory power and accuracy. R2 is a key statistical measurement used to evaluate how well the regression model fits the observed data. It indicates the proportion of variance in the dependent variable that is explained by the independent variables. A higher R2 value suggests that the model explains a large portion of the variability in the response variable, whereas a lower R2 value indicates a less effective model fit. The coefficient of determination is calculated as:
- Where SSres is the residual sum of squares: ∑(y -ŷ)2
- SStot is the total sum of squares: ∑(y -ȳ)2
y represents the observed values, ŷ denotes the predicted values, and ȳ is the mean of the observed values.
This measurement measures the proportion of variance in the dependent variable that is explained by the independent variables in the regression model. By conducting this statistical analysis, the research team was able to develop a robust predictive model that accurately forecasts A. mellifera overwintering mortality based on key climatic factors.
RESULTS
1. Evaluating predictive models for Apis mellifera winter mortality rate
The ANOVA results demonstrate that the regression model for predicting Apis mellifera winter mortality rate is statistically significant. With an F-statistic of 58.108 and a p-value of 0.000, the model is significant at the 0.05 level, indicating that the independent variables, excluding the Spring to Autumn Optimal Climate Index days, collectively explain a considerable portion of the variance in winter mortality rate. The total sum of squares was 6,382.977, with a regression sum of squares of 5,644.706 and a residual sum of squares of 738.272. These values suggest that the regression model provides a good fit to the data, offering meaningful insights into the determinants of A. mellifera overwintering mortality.
The regression coefficients reveal the individual predictors’ contributions to winter mortality rate (Table 3). The intercept indicates the baseline level of the winter mortality rate when all predictors are zero. The variable representing autumn low-temperature days has a significant negative impact, suggesting that more low-temperature days during autumn are associated with a reduced winter mortality rate. This aligns with the notion that gradual cooling stimulates winter cluster formation and fosters energy-conserving behaviors, ultimately enhancing colony survival (Becsi et al., 2021). In contrast, autumn high-temperature days and autumn precipitation days are not statistically significant, indicating no substantial influence on winter mortality rate. Winter low-temperature days, however, have a highly significant positive effect, with more low-temperature days in winter strongly linked to increased A. mellifera mortality (Switanek et al., 2016). This finding is consistent with previous research, which indicates that prolonged exposure to cold forces colonies to expend substantial energy on thermoregulation, thereby depleting their resources and heightening their susceptibility to disease and parasites (Maes et al., 2021). The coefficient for winter high-temperature days was found not to be statistically significant, suggesting that the impact of warm spells during winter on A. mellifera overwintering mortality may have been mitigated by regional climatic variations or beekeeping practices, such as artificial feeding and hive insulation. However, this result could be influenced by the fact that the model was constructed based solely on 2022 data. To better understand the relationship between winter high temperatures and winter mortality rate, further research with multi-year data collection and a comprehensive analysis of regional beekeeping practices would be necessary. The final regression equation for predicting A. mellifera overwintering mortality is:
- Where: Ŷ=Predicted overwintering mortality rate
- WF4=Autumn low-temperature days
- WF5=Autumn high-temperature days
- WF6=Autumn precipitation days
- WF7=Winter low-temperature days
- WF8=Winter high-temperature days
The validation of the model for A. mellifera winter mortality rate was conducted using the 2023 observed data on A. mellifera winter mortality rate (Fig. 2). The statistical measurements used for the validation included the coefficient of determination (R2), Mean Absolute Error (MAE), and Root Mean Squared Error (RMSE).
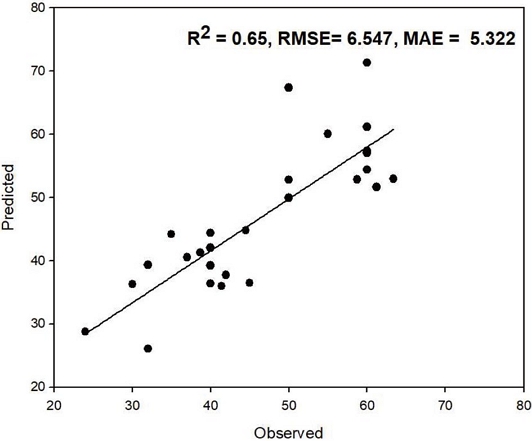
Model validation of Apis mellifera winter mortality predictions based on 2023 observed data.The scatter plot validates the model’s ability to predict A. mellifera winter mortality rate. It demonstrates a coefficient of determination of 0.65, indicating that the model explains 65% of the variance in the observed data. The Mean Absolute Error of 5.322 and Root Mean Squared Error of 6.547 suggests the model has moderate prediction accuracy, although some deviations and potential outliers appear to affect the error measurements.
The model achieved an R2 of 0.65, indicating that 65% of the variance in the observed data is explained by the model. This suggests a moderately strong relationship between the predicted and observed values, though 35% of the variance remains unexplained, pointing to areas for further improvement.
The MAE of 5.322 shows that, on average, the absolute deviation between predicted and observed losses is approximately 5.3 units. Similarly, the RMSE of 6.547 indicates the average magnitude of prediction errors, meaning the model’s predictions deviate from the observed values by about 6.5 units on average. The higher RMSE compared to MAE suggests the presence of outliers or a few cases with larger deviations, which influence the error measurements.
The scatter plot demonstrates a general alignment between the predicted and observed values, with most data points closely following the regression line. However, some deviations, particularly at higher loss values, indicate that the model struggles to accurately predict extreme cases. The presence of outliers suggests that additional factors, such as regional environmental differences, management practices, or disease prevalence, may influence A. mellifera winter mortality rate beyond the scope of the current model. Despite these limitations, the model’s overall performance provides a reasonably reliable tool for predicting A. mellifera overwintering mortality based on key climatic variables. This suggests that this model can be applied to simulate the impact of climate change on A. mellifera winter mortality rate.
2. Temporal analysis of climate change impacts on Apis mellifera ecosystems
This study examines the projected changes in climate and overwintering bee ecosystems in South Korea based on climate change scenarios for 2030, 2050, and 2100. The methodology involved generating daily raster layers of maximum, minimum, and mean temperature through interpolation methods using the daily data from the climate change scenarios. These time points serve as significant benchmarks for international climate policies and research, representing short-term, mid-term, and longterm perspectives. Evaluating these time frames is essential for assessing the effectiveness of climate change response strategies and policies, as well as developing future adaptation plans (Parson and Karwat, 2011). Specifically, the 2030 scenario is suitable for examining the initial effects of current climate policies, 2050 is an important milestone for achieving carbon neutrality and preparing mid-term adaptation strategies, and 2100 marks the endpoint for long-term climate change, allowing for an assessment of the extreme impacts of global warming on ecosystems and biodiversity (Randerson et al., 2015).
Fig. 3 illustrates the projected changes in average air temperature across South Korea, highlighting a significant warming trend from 2030 to 2100. By 2030, the nation exhibits a cool-toned gradient, with average temperatures ranging from approximately -19℃ in the northern and mountainous regions to near 0℃. By 2050, the warming intensifies, with the prevalence of green and yellow zones indicating moderate temperature increases. By 2100, the warming trend peaks, with the map predominantly shaded in red tones, signifying widespread average temperatures around 21℃. This progression underscores the critical impact of climate change on A. mellifera overwintering ecosystems in South Korea. The drastic increase in annual air temperatures projected for the country has the potential to significantly alter the habitats and survival of A. mellifera colonies, with far-reaching consequences for ecological systems and agricultural pollination (Cho et al., 2021).
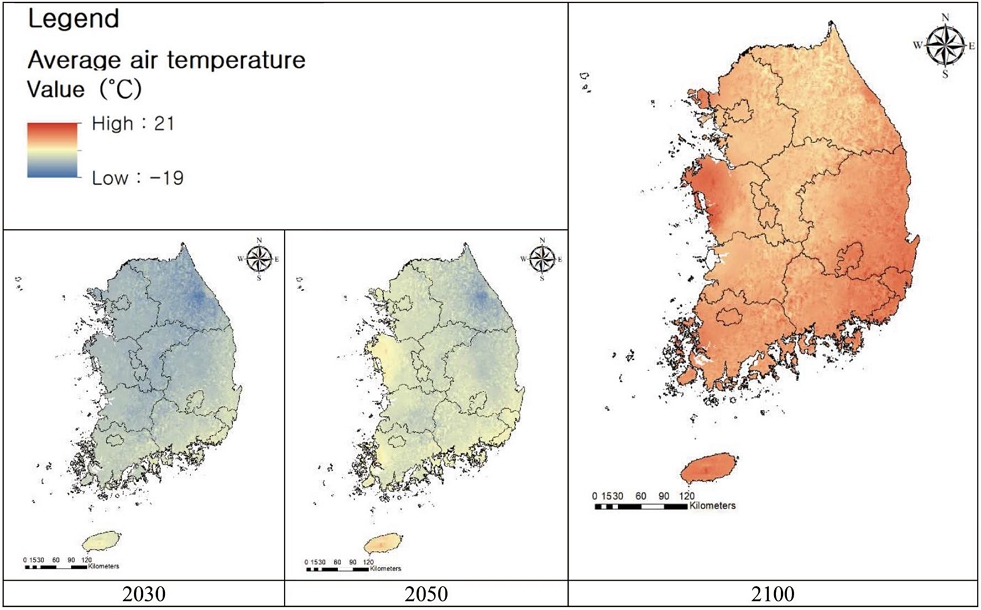
Future climate projections: average air temperature changes in South Korea (2030, 2050, 2100).The figure depicts projected average air temperature changes across South Korea for 2030, 2050, and 2100, showing a clear warming trend over time. By 2100, most regions are expected to experience significantly higher temperatures, with averages ranging from 15℃ to 21℃, highlighting the potential impact of climate change on ecosystems, including Apis mellifera overwintering habitats.
The regional climate projections for South Korea from 2030 to 2100 reveal distinct spatial patterns. In 2030, northern regions, including high-altitude areas like Gangwon Province, are expected to remain relatively cooler, with average temperatures around –19℃. Conversely, southern regions such as Jeollanam-do and Gyeongsangnam-do exhibit comparatively warmer climates, ranging from -5℃ to 0℃. By 2050, central South Korea experiences a moderation of temperature gradients, with most areas transitioning to a range of -5℃ to 10℃. While high-altitude regions like Gangwon Province continue to experience relatively cooler climates, a discernible warming trend is observed compared to 2030. Notably, southern coastal areas, including Busan and Jeju Island, exhibit significant warming, with averages approaching 15℃. By 2100, the impacts of climate change become starkly evident on a nationwide scale, with the majority of the country projected to experience average annual temperatures between 15℃ and 21℃. The historically coldest northern mountainous regions undergo unprecedented warming, with average temperatures nearing 10℃ to 15℃. Furthermore, urban and coastal areas, particularly in the southern and western regions, including metropolitan areas such as Seoul, Busan, and Jeju Island, display the highest temperatures, often exceeding 20℃.
Fig. 4 illustrates the projected changes in maximum air temperature across South Korea, highlighting a significant warming trend from 2030 to 2100. By 2030, the maximum temperatures are expected to range from around -2.7℃ in the colder northern and high-altitude areas to 5-10℃ in the southern and coastal regions. This moderate warming pattern is indicated by the dominance of blue tones in the north and yellowish tones in the south, suggesting the beginning of a shift towards a warmer climate. By 2050, the warming trend becomes more pronounced, with maximum temperatures increasing to 5 over 10℃ across most areas. Distinct hotspots emerge, particularly in the southern coastal regions and Jeju Island, where maximum temperatures approach 15-18℃. These areas, depicted in orange and red, are among the first to experience significant climate shifts, aligning more closely with subtropical patterns. This highlights a growing disparity between the relatively cooler northern regions and the increasingly warm southern and coastal areas. By 2100, the warming intensifies dramatically, with maximum air temperatures predominantly ranging from 15 to 18.4℃ across South Korea. The map transitions to an almost entirely red color, indicating a substantial and widespread increase in maximum temperatures. Even the historically cooler northern and high-altitude regions experience unprecedented warming, with maximum temperatures reaching 10-15℃. The urban and coastal areas, including major metropolitan regions, exhibit extreme maximum temperatures nearing or exceeding 18℃, becoming the hottest parts of the country by the end of the century.
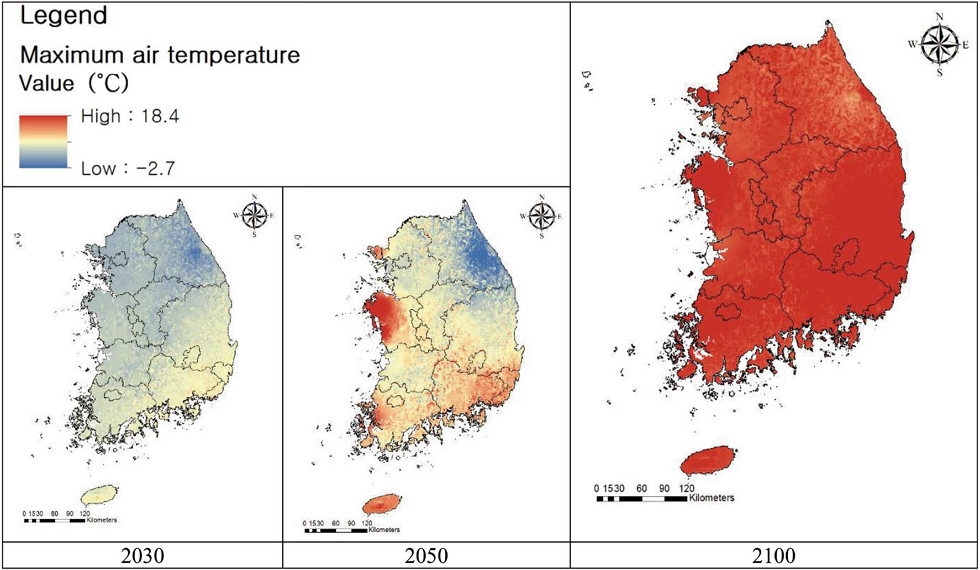
Future climate projections: maximum air temperature changes in South Korea (2030, 2050, 2100).The figure illustrates projected changes in maximum air temperatures across South Korea from 2030 to 2100, revealing a significant warming trend. By 2100, maximum temperatures predominantly range from 15℃ to 18.4℃ nationwide, with southern and coastal areas experiencing the most pronounced warming
The regional climate projections for South Korea from 2030 to 2100 reveal distinct spatial patterns in maximum temperatures that warrant a detailed analysis. In 2030, northern regions, including mountainous areas like Gangwon Province, are projected to maintain relatively low maximum temperatures around -2.7℃. Central and southern regions, such as Gyeonggi Province and Gyeongsang-do, exhibit moderate warming with maximum temperatures ranging from 5℃ to 10℃. Southern coastal areas and Jeju Island show slightly higher maximum temperatures, nearing 10℃, reflecting early warming impacts. By 2050, northern high-altitude areas see a significant temperature rise, ranging between 0℃ and 10℃, though remaining cooler than the rest of the country. Central and southern regions experience a noticeable increase, with maximum temperatures consistently ranging from 10℃ to 15℃. Southern coastal areas and Jeju Island undergo a sharper rise, with maximum temperatures nearing 15℃ to 18℃, indicating substantial warming compared to 2030. By 2100, the impact of warming is evident nationwide. Maximum temperatures across most of the country range between 15℃ and 18.4℃, with northern and high-altitude regions climbing to unprecedented levels of 10℃ to 15℃. Urban and coastal hotspots, such as Seoul, Busan, and Jeju Island, face maximum temperatures exceeding 18℃, making them the warmest regions in South Korea.
The minimum temperature is also expected to rise continuously from 2030 to 2100 (Fig. 5). By 2030, the minimum air temperatures are projected to range from approximately -11.4℃ in the colder northern and high-altitude regions to near 0℃ in southern and coastal areas. This moderate warming is visually depicted by the dominance of blue tones in northern areas, while lighter yellow tones emerge in the southern regions, indicating slightly warmer minimum temperatures compared to the current baseline. By 2050, minimum temperatures are expected to rise noticeably across most parts of the country, ranging from -5℃ in northern regions to 10℃ in southern areas. Notably, southern coastal regions and Jeju Island exhibit a sharper warming trend, with minimum temperatures approaching 10℃ to 15℃. The increasing contrast between the colder northern mountainous areas and the warming southern lowlands highlights the intensifying warming trend. By 2100, the warming becomes dramatic, with minimum temperatures predominantly ranging between 10℃ and 16.3℃ nationwide. The map transitions into a mix of yellow and red tones, signaling widespread and substantial warming across the entire country. Northern regions, which historically experienced freezing minimum temperatures, undergo significant warming, with minimum temperatures reaching between 5℃ and 10℃. Meanwhile, southern and urban areas, such as Busan and Jeju Island, record the highest minimum temperatures, exceeding 15℃, reflecting the most pronounced warming trends across South Korea.
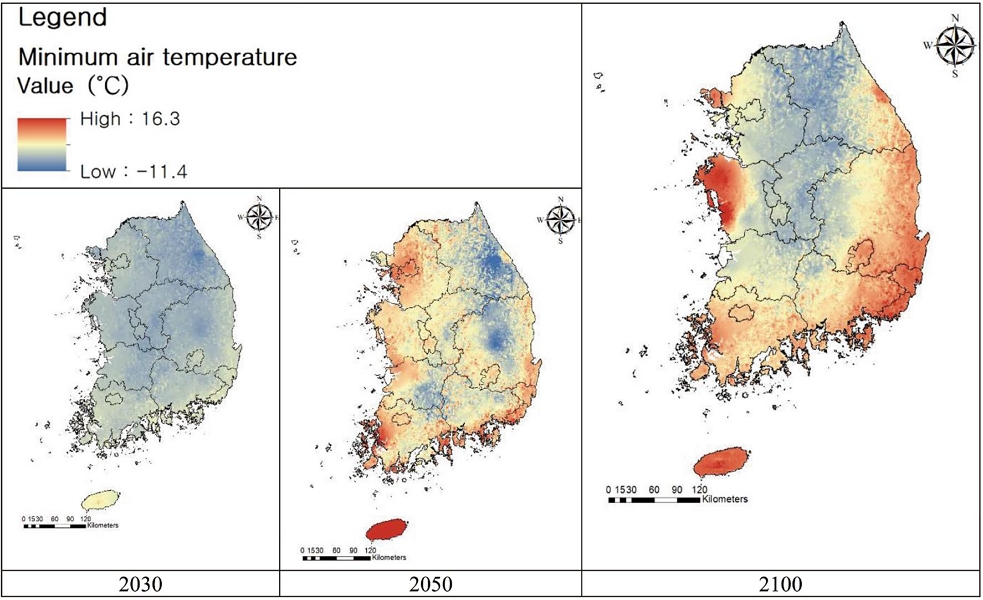
Future climate projections: minimum air temperature changes in South Korea (2030, 2050, 2100).The figure illustrates the projected rise in minimum air temperatures across South Korea from 2030 to 2100, with a significant warming trend. By 2100, minimum temperatures are expected to range from 10℃ to 16.3℃ nationwide, with southern and coastal regions, such as Busan and Jeju Island, experiencing the most pronounced increases
The regional climate projections for South Korea from 2030 to 2100 reveal distinct spatial patterns in minimum temperatures that warrant a detailed analysis. In the 2030 projections, northern regions, including high-altitude areas like Gangwon Province, are expected to maintain low minimum temperatures around -11.4℃. In contrast, central and southern regions, such as Gyeonggi Province and Gyeongsang-do, exhibit moderate warming, with minimum temperatures ranging from -5℃ to 0℃. Jeju Island and southern coastal areas show slightly higher minimum temperatures, nearing 0℃, indicating early signs of warming in these regions. By 2050, northern high-altitude areas experience a rise in minimum temperatures, ranging between -5℃ and 0℃. Although these regions remain colder than the rest of the country, the warming trend is evident. In the central and southern regions, minimum temperatures increase significantly, ranging from 0℃ to 10℃, with widespread warming across the central plains. Southern coastal areas and Jeju Island see a marked increase in minimum temperatures, reaching 10℃ to 15℃, signaling a shift toward a more subtropical climate in these areas. By 2100, the minimum temperatures across northern and high-altitude regions, which historically experienced prolonged freezing conditions, rise substantially, ranging between 5℃ and 10℃. Central and southern regions also see minimum temperatures rise to 10℃ to 15℃, indicating widespread warming in areas that were previously cooler. Urban and coastal areas, including Busan and Jeju Island, experience the highest minimum temperatures, exceeding 15℃, making them the warmest regions in the country. These warming trends reflect the profound impact of climate change, necessitating targeted adaptation and mitigation strategies to address the challenges posed by such significant temperature increases.
The projected changes in maximum, minimum, and average temperatures across South Korea from 2030 to 2100 highlight the significant and widespread warming trends. Northern and high-altitude regions, traditionally known for their cooler climates, are expected to experience substantial warming, with average, maximum and minimum temperatures rising substantially. Central and southern regions, as well as coastal areas and Jeju Island, are projected to undergo even more pronounced warming, with average, maximum and minimum temperatures reaching unprecedented levels.
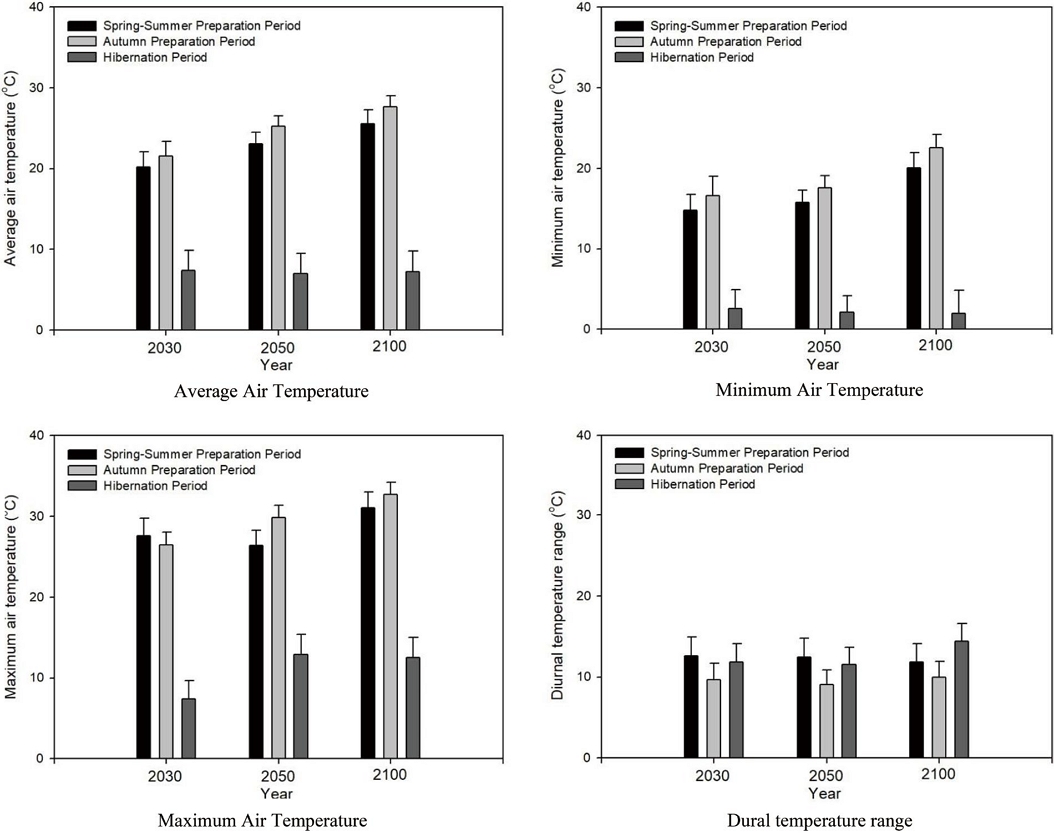
Future projected seasonal air temperature trends and diurnal temperature range for A. mellifera overwintering periods (2030, 2050, 2100).The figure shows projected trends in average, maximum, and minimum air temperatures, as well as diurnal temperature range (DTR), for the three seasonal periods crucial to A. mellifera overwintering. By 2100, significant warming is projected, with higher minimum and maximum temperatures reducing freezing conditions critical for overwintering. While DTR narrows during hibernation, it remains relatively stable in warmer seasons, highlighting challenges for bee survival during winter.
3. The impact of climate change on Apis mellifera ecosystem resilience
The study categorized the Apis mellifera overwintering periods into three distinct stages based on their activity and preparation for winter survival: the spring-autumn preparation period, the autumn preparation period, and the winter overwintering period. These stages represent the critical phases in the Apis mellifera annual cycle, each presenting unique climate-related challenges.
By 2030, projections indicate that average air temperatures will range from approximately 5℃ to 15℃ across different seasons, with the highest averages occurring during the spring-summer preparation period. During this time, maximum temperatures are expected to rise from around 20℃ during hibernation to roughly 30℃, while minimum temperatures will range from 0℃ during hibernation to 10℃ during warmer periods. The diurnal temperature range-the difference between daytime highs and nighttime lows-is projected to remain relatively stable, fluctuating between 10℃ and 15℃. However, the spring-summer preparation period may exhibit slightly higher variability, indicating more pronounced day-night temperature differences compared to other periods.
By 2050, average air temperatures are projected to rise further, ranging from 10℃ to 20℃, with the highest averages during the spring-summer preparation period. Maximum temperatures are likely to climb to between 25℃ and 35℃, while minimum temperatures are expected to increase from 5℃ to 15℃, particularly impacting traditionally cooler periods. The diurnal temperature range is expected to narrow slightly during hibernation due to rising minimum temperatures, reducing day-to-night variability. However, for other periods, the DTR is anticipated to remain relatively stable, fluctuating between 10℃ and 15℃.
By 2100, the warming trend is expected to accelerate, with nationwide average temperatures ranging between 15℃ and 25℃, and maximum temperatures surpassing 35℃ during the spring-summer preparation period. Minimum temperatures will also rise significantly, from 10℃ to 20℃, suggesting a substantial reduction in freezing conditions essential for A. mellifera overwintering. Despite these changes, the DTR for the spring-summer and autumn periods is expected to remain consistent, while narrowing further during hibernation. The error bars representing regional deviations suggest that disparities in temperature patterns will become more pronounced by the end of the century, with significant implications for A. mellifera survival and agricultural pollination.
Diurnal temperature range is a crucial parameter for analyzing seasonal climate dynamics, particularly in understanding its impact on ecological systems like A. mellifera populations (Sgolastra et al., 2011). A. mellifera colonies are highly sensitive to changes in temperature variability, especially during hibernation, when maintaining a stable internal hive temperature is crucial for their survival (Ricigliano et al., 2018). By 2030, the DTR across seasonal periods is projected to remain relatively stable, fluctuating between 10℃ and 15℃, with the highest values observed during the spring-summer period. In contrast, the hibernation period will have narrower DTR values, indicating more stable nighttime temperatures, which could potentially disrupt the bees’ natural wintering processes. By 2050, rising minimum temperatures are expected to further narrow the DTR during hibernation, reducing variability to around 10℃. This reduced thermal fluctuation may hinder the bees’ ability to regulate hive temperature and maintain their energy reserves. By 2100, the narrowing of DTR during hibernation is expected to continue, while the DTR for the spring-summer and autumn periods remains more stable, highlighting the resilience of day-night temperature patterns in warmer seasons but also underscoring the increasing challenge for A. mellifera during the winter months.
Regional variability in temperature patterns, as indicated by the standard deviations represented in the error bars, is expected to change significantly by 2100. In 2030, regional variability is expected to be relatively minor, with most areas in South Korea staying within 1-2℃ of the national average. By 2050, regional variability is projected to increase slightly, especially during the autumn preparation period, indicating the beginning of more pronounced regional differences in warming trends. By 2100, these differences are expected to become more significant, particularly during the spring-summer and autumn periods, with standard deviations widening to 3-5℃. This suggests that southern coastal and urban areas may experience greater temperature increases compared to northern and high-altitude regions, potentially affecting A. mellifera populations differently depending on their location.
The implications of these temperature trends, including DTR and regional variability, are profound for A. mellifera colonies and their ability to survive winter (Ricigliano et al., 2018). A narrowing DTR during the hibernation period could negatively impact A. mellifera colonies, which rely on cold nighttime conditions to slow their metabolism and conserve energy (Nürnberger et al., 2018). Reduced temperature variability may lead to increased metabolic activity, depleting energy reserves more quickly and resulting in higher winter mortality rates (Switanek et al., 2016). Additionally, agricultural crops that rely on A. mellifera pollination may face reduced yields due to declining bee populations, particularly in regions experiencing consistently narrower DTRs (Sgolastra et al., 2011; Calovi et al., 2021).
The increasing regional variability, as indicated by growing standard deviations, also highlights the need for tailored climate adaptation strategies (Millard et al., 2023). Southern coastal and urban areas, which are projected to experience the largest deviations, may require targeted interventions to address the compounded effects of heat stress and reduced overwintering success in bee populations. The projected temperature increases and changing DTR have critical implications for the survival of A. mellifera colonies during winter. Accelerated warming during the spring-summer preparation period could disrupt biological processes, such as foraging behavior and colony growth, by altering the timing of flowering and resource availability (Johannesen et al., 2022). The autumn preparation period, essential for building energy reserves for winter, may benefit from extended foraging opportunities but also risks disrupting brood production and hive thermoregulation (Seeley and Visscher, 1985; Mattila and Otis, 2007). The hibernation period, however, remains the most vulnerable, as a narrowing diurnal temperature range could compromise the bees’ ability to regulate hive temperature and conserve energy, potentially leading to higher winter mortality rates (Sgolastra et al., 2011). With average temperatures rising to between 15℃ and 20℃, and maximums reaching 30℃, A. mellifera colonies may struggle to enter a proper state of dormancy, leading to increased energy expenditure, reduced survival rates, and potential colony collapse (Calovi et al., 2021). These changes underscore the importance of understanding not only absolute temperature increases but also variability within daily cycles, as these factors directly influence A. mellifera physiology, colony survival, and broader ecological resilience (Bordier et al., 2017). The analysis of diurnal temperature ranges and regional standard deviations emphasizes the complexity of seasonal climate change impacts, highlighting uneven effects across regions.
4. Assessing the impacts of climate change on Apis mellifera overwintering in Korea using climate indices
This study developed climate-based indices to examine the impact of climate change on Apis mellifera overwintering and mortality in Korea, analyzing scenarios for 2030, 2050, and 2100.
Fig. 7 shows that analyzing the effects of climate change on the Spring to Autumn Optimal Climate Index in South Korea highlights significant shifts in the duration of optimal conditions for bee activity over the decades. The Autumn Optimal Climate Index, which represents the duration of favorable climate conditions for bee survival and activity, reveals a gradual increase over time.
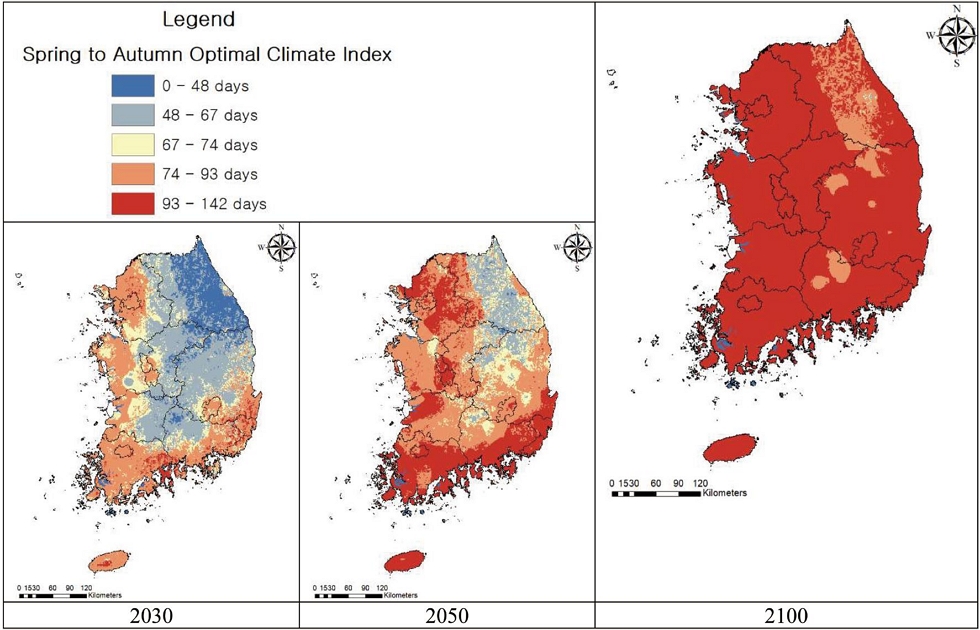
Projected changes in spring to autumn optimal climate index in South Korea (2030, 2050, 2100).The figure illustrates the projected increase in the Spring to Autumn Optimal Climate Index across South Korea from 2030 to 2100. By 2100, most regions, particularly in southern and coastal areas, are expected to experience prolonged optimal conditions for A. mellifera activity, with durations exceeding 93 days, reflecting a significant shift due to climate change.
Between 2030 and 2100, the Spring to Autumn Optimal Climate Index across South Korea shows a clear trend of prolonged optimal climate conditions, particularly in the southern and coastal regions. By 2030, northern and mountainous areas, such as Gangwon Province, maintain a relatively short Spring to Autumn Optimal Climate Index of less than 48 days, indicating limited suitability for extended bee activity. In contrast, southern coastal regions, including Jeolla and Gyeongsang Provinces, exhibit a more favorable Spring to Autumn Optimal Climate Index, exceeding 74 days. The central regions, such as Gyeonggi and Chungcheong Provinces, experience intermediate durations of around 48-67 days, suggesting moderate conditions for bee activity.
By 2050, climate models predict broader suitability across South Korea. The southern and coastal areas continue to lead with Spring to Autumn Optimal Climate Index durations exceeding 74 days, while the central regions begin to reach similar levels of suitability. Even the northern areas, which previously had shorter durations, show an increase to around 48-67 days. This northward expansion of optimal climate conditions reflects the warming trends associated with climate change, suggesting improved conditions for pollinator activity in regions previously constrained by cooler climates.
The year 2100 marks the most significant expansion of Spring to Autumn Optimal Climate Index across South Korea, with most regions exhibiting durations exceeding 93 days. The central and southern areas maintain optimal conditions for as long as 93-142 days, and even the northern provinces, including Gangwon, experience notable increases, with Spring to Autumn Optimal Climate Index durations ranging from 67 to 74 days. This indicates a comprehensive shift toward longer active periods for bees across the entire South Korea.
The increase in Spring to Autumn Optimal Climate Index across most regions of South Korea indicates that climate change is leading to more favorable conditions for A. mellifera overwintering. The prolonged duration of optimal climate conditions, particularly in the southern and coastal areas, suggests that bees will have extended foraging periods and better opportunities to accumulate the necessary energy reserves for successful overwintering.
Analyzing the impact of climate change on autumn low-temperature conditions in South Korea is critical, as a decline in low-temperature days could pose a significant threat to the preparation of Apis mellifera populations for successful overwintering. This examination, within the climate change scenarios, focuses on the availability of days with sufficiently low temperatures necessary for A. mellifera overwintering preparation, as shown in Fig. 8.
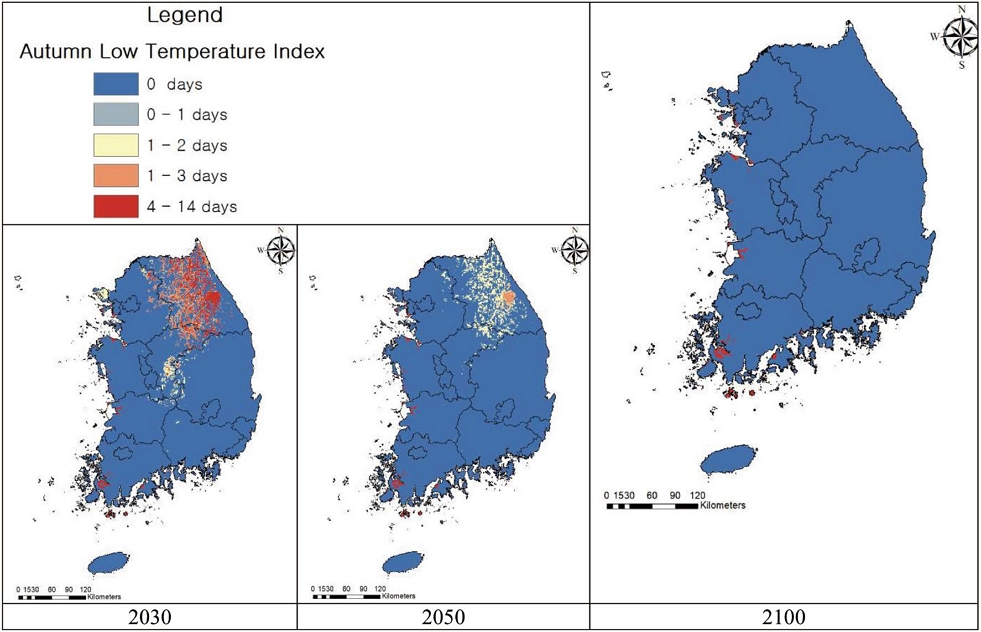
Projected decline in autumn low-temperature days in South Korea (2030, 2050, 2100).The figure depicts the projected decline in autumn low-temperature days across South Korea from 2030 to 2100. By 2100, the majority of the country, including historically cooler northern regions, is expected to experience minimal to no low-temperature days, posing significant challenges to A. mellifera overwintering preparation.
The findings indicate a concerning decline in low-temperature days across South Korea due to climate change. Projections suggest that by 2100, most of the country will experience conditions unsuitable for effective A. mellifera overwintering preparation, posing a significant threat to their survival. From 2030 to 2100, the number of low-temperature days is predicted to decrease dramatically.
In 2030, northern regions, such as northern Gyeonggi and northern Gangwon provinces, retain relatively favorable conditions, with some areas experiencing 4 to 14 low-temperature days. These less climate-impacted areas still provide an adequate environment for A. mellifera to prepare for overwintering. However, central and southern regions see a significant reduction in low-temperature days. Southern coastal areas, including Jeollanam-do and Gyeongsangnam-do, already exhibit fewer than one day of low temperatures by 2030, indicating an early onset of climate-driven challenges for A. mellifera overwintering.
By 2050, the situation worsens across the country, with the number of low-temperature days declining further. Northern regions such as Gangwon Province show only 1 to 2 days of low temperatures, while central and southern areas face near-total elimination of such conditions. In southern coastal regions like Busan, Ulsan, and Jeju, low-temperature days are virtually nonexistent. This trend highlights the widening regional gap in supporting A. mellifera populations during the critical overwintering preparation phase.
By 2100, the projections are even more concerning, as low-temperature days are expected to vanish almost entirely across the Korean Peninsula. Northern areas, which had previously maintained some resilience, are now predicted to experience only sporadic occurrences of 0 to 1 low-temperature days. Meanwhile, central and southern regions face a complete absence of these critical conditions. This lack of low-temperature days in southern coastal regions, such as Jeollanam-do and Gyeongsangnam-do, will severely hinder A. mellifera overwintering preparation, leading to potentially catastrophic declines in bee populations.
The drastic decline in low-temperature days disrupts A. mellifera ability to properly enter an overwintering phase. This compromises their capacity to accumulate adequate energy reserves and effectively regulate their body temperatures (Rajagopalan et al., 2024). Consequently, the risk of colony failure during the winter months is heightened. Southern and coastal regions are particularly vulnerable, as the complete loss of low-temperature days in these areas will prevent A. mellifera from undergoing the critical physiological processes required for winter survival (Ricigliano et al., 2018). Even northern regions, which still retain some low-temperature days, are expected to face increasing challenges as these days become fewer and more sporadic.
Fig. 9 investigates the effects of climate change on the number of high-temperature days experienced during the autumn season, as reflected in the Autumn High Temperature Index. This index measures the frequency of high-temperature days in the crucial autumn period when Apis mellifera colonies prepare for overwintering. Prolonged exposure to elevated temperatures can disrupt the bees’ ability to accumulate the essential energy reserves needed to survive the winter.
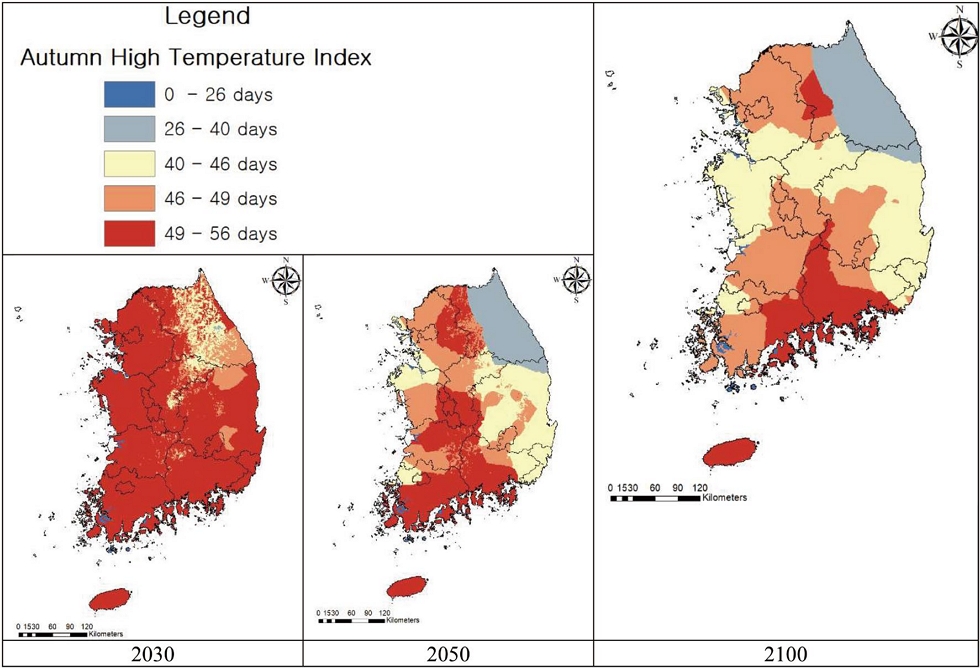
Projected autumn high temperature index across South Korea (2030, 2050, 2100).The figure shows the projected increase in high-temperature days during autumn in South Korea, highlighting the Autumn High Temperature Index from 2030 to 2100. Southern and central regions are expected to experience the most significant increases, with over 49 high-temperature days by 2100, disrupting A. mellifera overwintering preparations and raising the risk of colony failure.
From 2030 to 2100, the study reveals a clear upward trend in high-temperature days across South Korea, with the most dramatic increases observed in southern and central regions. By 2030, most areas experience over 46 high-temperature days, with southern regions such as Jeollanam-do and Gyeongsangnam-do reaching 49 to 56 days, the highest in the country. These extended high-temperature conditions are likely to hinder A. mellifera colonies from adequately transitioning into overwintering phases, leaving them unable to accumulate the necessary energy reserves for winter survival. Northern and mountainous regions, including northern Gangwon Province, exhibit slightly fewer high-temperature days, ranging from 40 to 46 days. However, even these levels are elevated compared to historical norms, indicating the widespread impact of climate warming.
By 2050, the number of high-temperature days decreases slightly in some northern areas, such as northern Gyeonggi and northern Gangwon provinces, where they range from 26 to 40 days. These regions may provide relatively better conditions for A. mellifera overwintering preparation compared to southern and central areas. However, in southern regions, including Jeollanam-do and Gyeongsangnam-do, high-temperature days remain high, exceeding 49 days in many areas. This persistent trend underscores the heightened vulnerability of southern regions to climate-driven challenges, as the prolonged high-temperature conditions limit the time available for A. mellifera colonies to prepare for winter.
By 2100, high-temperature days are projected to remain elevated across much of South Korea, with southern and central regions continuing to face the most significant challenges. Areas such as Jeollanam-do and Gyeongsangnam-do are expected to experience over 49 high-temperature days, creating highly unfavorable conditions for A. mellifera overwintering. In contrast, northern regions, including northern Gangwon and northern Gyeonggi provinces, show a slight reduction in high-temperature days, ranging from 26 to 40 days. While these northern areas may retain some capacity to support A. mellifera overwintering, they are still significantly affected by warming trends and higher temperatures compared to historical averages.
The rise in high temperatures during autumn disrupts A. mellifera colonies’ ability to prepare for winter (Calovi et al., 2021). This leads to insufficient energy reserves and increases the risk of colony failure during the winter months (Switanek et al., 2016). The effects are particularly severe in southern and coastal regions of South Korea, where high temperatures are most prolonged. While northern regions are less affected, they still face challenges due to climate change. The extended exposure to high temperatures in autumn prevents the bees from properly transitioning into the overwintering phase. This disrupts their physiology and prevents them from accumulating necessary energy reserves (Salehizadeh et al., 2020). This raises the likelihood of significant colony losses, especially in southern and coastal areas where high temperatures are most pronounced. The northern regions may be relatively less affected, but they too face heightened challenges from the widespread impacts of climate change.
This study examines the impact of climate change on autumn precipitation patterns, with a focus on the Autumn Precipitation Index. This index measures the number of rainy days during the crucial autumn season. Rainy days during this critical period can significantly disrupt A. mellifera activity, hindering their ability to forage and accumulate the essential energy reserves needed for overwintering (Johannesen et al., 2022). Projections indicate that, due to climate change, the number of autumn precipitation days is expected to rise across South Korea, particularly in the southern and central regions, by the year 2100 (Fig. 10).
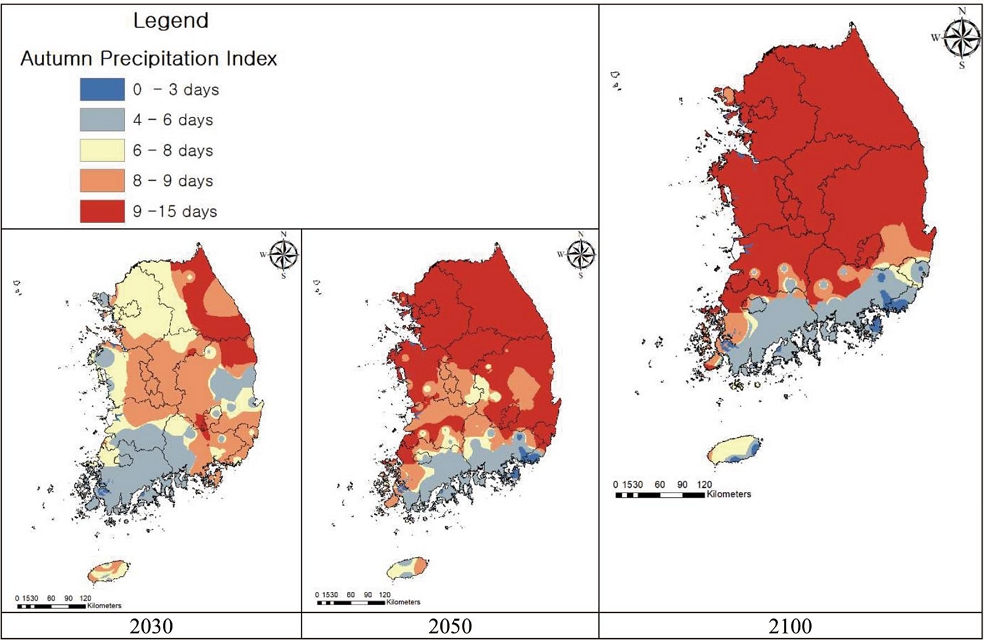
Projected autumn precipitation index across South Korea (2030, 2050, and 2100).The figure illustrates the projected increase in autumn precipitation days across South Korea from 2030 to 2100. By 2100, southern and central regions are expected to experience the highest levels of rainfall, with 9 to 15 rainy days, significantly disrupting A. mellifera foraging and overwintering preparation.
By 2030, northern regions like Gangwon and Gyeonggi exhibit relatively low precipitation, with only 0 to 3 rainy days. These areas may provide more favorable conditions for Apis mellifera to prepare for winter. However, central regions such as Chungcheong and Jeolla see a rise to 4 to 6 rainy days, presenting moderate challenges to A. mellifera activity. Meanwhile, southern regions including Gyeongsangnam-do and Jeollanam-do are already experiencing higher precipitation levels of 8 to 9 rainy days. This early increase in rainfall disrupts A. mellifera foraging and preparation, making these southern areas particularly vulnerable to climate change effects.
By 2050, autumn precipitation continues to rise across much of the country. Most regions, including central areas, see 6 to 8 rainy days, further limiting the time A. mellifera have to forage and store energy. Northern regions like Gyeonggi and Gangwon still retain lower precipitation of 4 to 6 rainy days, providing comparatively better overwintering conditions. However, southern coastal regions including Gyeongsangnam-do, Jeollanam-do, and parts of Chungcheongnam-do experience a persistent 8 to 9 rainy days. This prolonged rainfall severely restricts A. mellifera activity and energy storage, increasing the risk of colony stress and overwintering failure.
By 2100, the impact on autumn precipitation is even more pronounced. Most regions see 8 to 9 rainy days, with southern and central areas facing the highest levels of 9 to 15 days. Southern coastal regions like Jeollanamdo and Gyeongsangnam-do are particularly affected, experiencing the country’s highest precipitation. These conditions are expected to significantly hinder A. mellifera activity, making it nearly impossible for colonies to gather sufficient resources for overwintering (Kim et al., 2023a). While northern regions like Gangwon and Gyeonggi see relatively fewer 4 to 6 rainy days, they too are impacted by the overall trend of increased precipitation, which could reduce ecosystem stability over time. Increased rainfall during the autumn months impedes A. mellifera ability to forage efficiently, diminishing their capacity to accumulate the energy reserves necessary for overwintering (Switanek et al., 2016). Regions with the highest autumn precipitation, especially in southern and central South Korea, face the greatest risk of A. mellifera colony losses due to inadequate resource collection for overwintering. Even the relatively stable northern regions are not exempt from the effects of climate change.
This study also examines the impact of climate change on winter low-temperature days, a critical factor for Apis mellifera overwintering survival. The Winter Low-Temperature Index quantifies the number of days with sufficiently low temperatures during winter, which are essential for A. mellifera colonies to conserve energy and maintain their winter clusters. Cold weather during winter helps A. mellifera reduce energy consumption by remaining in a dormant state, ensuring they have enough reserves to survive until spring (Becsi et al., 2021). Between 2030 and 2100, the study highlights a progressive decline in the number of low-temperature days across South Korea, with southern and coastal regions being the most severely affected (Fig. 11).
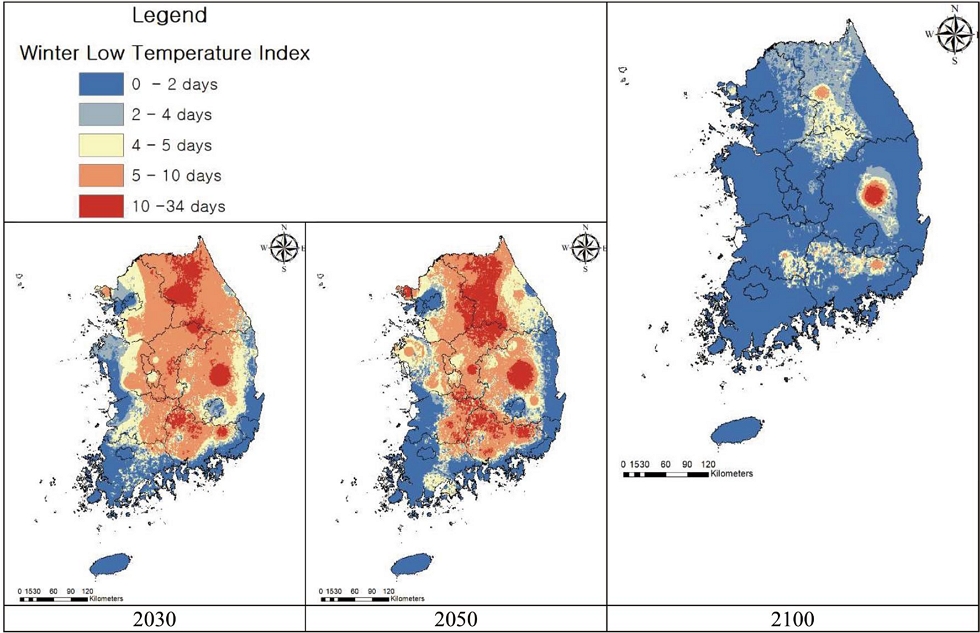
Projected winter low-temperature index across South Korea (2030, 2050, 2100).The figure highlights the projected decline in winter low-temperature days across South Korea from 2030 to 2100. By 2100, most regions, especially southern and coastal areas, experience near-total loss of low-temperature days
By 2030, areas such as Chungcheong-do and parts of Gyeonggi-do experience 5 to 10 low-temperature days, providing moderately favorable conditions for A. mellifera overwintering. Some regions in Jeonbuk and Gyeongbuk also have 4 to 5 days of sufficiently low temperatures, offering marginally suitable conditions for A. mellifera colonies. However, southern coastal regions, including Jeollanam-do and Gyeongsangnam-do, already exhibit a significant reduction in low-temperature days, with many areas recording only 0 to 2 days. This early onset of warming in the south poses immediate challenges for A. mellifera overwintering preparation.
By 2050, the conditions continue to worsen across much of the country. The number of low-temperature days across the country decreases to just 2 to 4 days, reflecting a narrowing window of suitable conditions for A. mellifera overwintering. Northern areas such as northern Gyeonggi-do and northern Gangwon-do retain slightly more favorable conditions, with 4 to 5 low-temperature days, but even these regions show a declining trend. In southern and coastal regions, including southern Gyeongsang-do and Jeollanam-do, low-temperature days almost disappear entirely, with most areas recording only 0 to 2 days. This decline severely limits the ability of A. mellifera colonies in these regions to prepare for winter, increasing their vulnerability to overwintering failure (Kim et al., 2023b).
By 2100, the decline in low-temperature days becomes critical. Most of South Korea experiences just 0 to 2 low-temperature days during winter, a near-complete loss of suitable conditions for A. mellifera overwintering. Only a few areas in northern Gangwon-do and northern Gyeonggi-do retain 2 to 4 low-temperature days, offering limited pockets of favorable conditions. Southern and coastal regions, such as Jeollanam-do and southern Gyeongsangdo, record virtually no low-temperature days, placing A. mellifera colonies in these areas under severe stress. The lack of sufficient cold weather forces A. mellifera to remain active throughout the winter, leading to increased energy expenditure, depleted reserves, and heightened risks of colony collapse (Ricigliano et al., 2018).
Without sufficient low-temperature days, A. mellifera colonies are unable to enter a proper dormant state, resulting in higher energy consumption and reduced survival rates during the winter (Johannesen et al., 2022). The progressive decline in low-temperature days across South Korea, particularly in southern and coastal regions, poses a severe threat to the overwintering success of A. mellifera colonies.
Fig. 12 examines the rising number of high-temperature days during winter due to climate change and the potential impact on Apis mellifera colonies. The Winter High Temperature Index quantifies the frequency of warm days in winter, which directly affects A. mellifera activity and energy consumption. Elevated winter temperatures can compel A. mellifera colonies to become more active, significantly increasing their energy expenditure and reducing their ability to conserve resources for survival (Johannesen et al., 2022). From 2030 to 2100, winter high-temperature days are projected to increase across all regions of South Korea, with the most substantial increases observed in the central and southern areas.
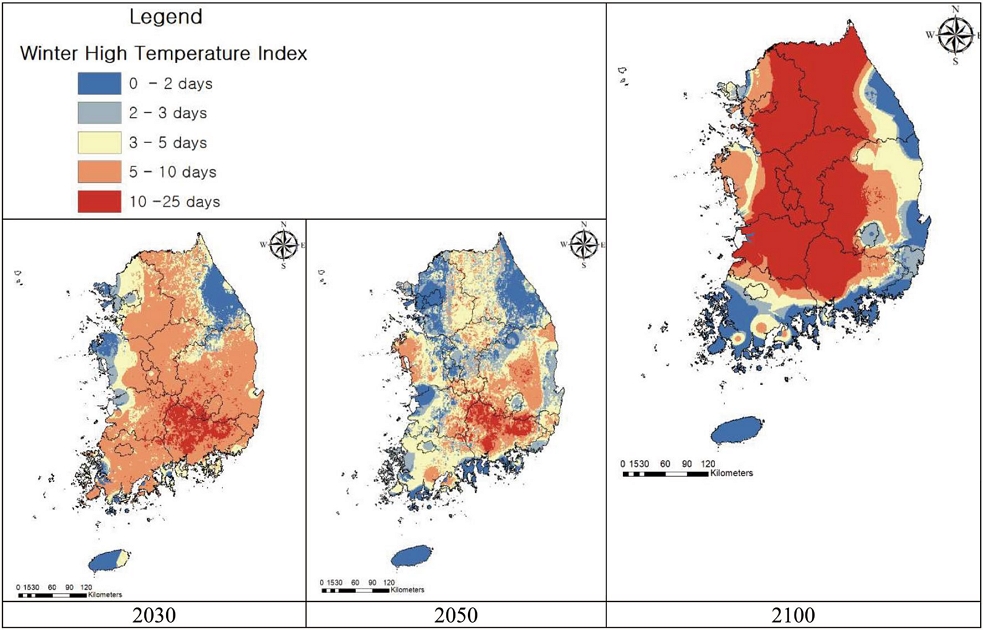
Projected winter high temperature index across South Korea (2030, 2050, 2100).The figure depicts the projected rise in winter high-temperature days across South Korea from 2030 to 2100, with the central and southern regions experiencing the most significant increases. By 2100, these regions face up to 25 high-temperature days
By 2030, areas such as southern Gyeonggi-do, Chungcheong-do, Jeolla-do, and inland Gyeongsang-do experience between 5 to 10 high-temperature days, while some southern inland regions record 10 to 25 such days. These extended warm periods disrupt A. mellifera overwintering by prompting unnecessary activity, depleting the critical energy reserves they need to survive. In contrast, northern regions like northern Gangwon-do and parts of northern Gyeonggi-do have relatively few high-temperature days (0-2), providing more stable conditions for A. mellifera overwintering.
By 2050, the situation worsens, with the central and southern inland regions experiencing a significant increase in high-temperature days. In Chungcheongnam-do, Gyeongsangbuk-do, and parts of Jeollanam-do, the number of high-temperature days rises to between 10 and 25, further straining A. mellifera colonies in these areas. In contrast, the northernmost regions, including northern Gangwon-do, maintain lower levels of high-temperature days (0-2), continuing to provide comparatively better conditions for overwintering. However, the general trend indicates an expansion of high-temperature conditions into previously cooler areas, signaling the widespread impacts of climate change across the Korean Peninsula.
By 2100, high-temperature days will be prevalent across most of South Korea. Regions such as Chungcheong-do, Gyeonggi-do, Jeolla-do, and Gyeongsang-do are projected to experience 10 to 25 high-temperature days, posing a critical challenge for A. mellifera overwintering survival. These extended warm periods will make it extremely difficult for A. mellifera colonies to conserve energy, leading to increased mortality rates and potential colony collapse (Maes et al., 2021). Even northern areas like northern Gangwon-do, which previously had more stable winter conditions, are expected to see a rise in high-temperature days, underscoring the pervasive effects of climate change. Southern and inland regions are particularly vulnerable, as their high-temperature days are anticipated to far exceed the thresholds needed for successful A. mellifera overwintering.
The increase in high-temperature days during winter forces A. mellifera to remain active, depleting their stored energy and reducing their chances of surviving until spring. This trend poses a significant threat to A. mellifera populations, particularly in the central and southern regions where the frequency of warm days is highest (Calovi et al., 2021). The disparity between regions highlights the urgent need for region-specific adaptive strategies to mitigate these effects.
5. Assessing the impacts of climate change on Apis mellifera overwintering in Korea
Fig. 13 shows the impact of climate change on Apis mellifera ecosystems in South Korea, focusing specifically on the winter mortality rates of A. mellifera colonies. The projected changes in the frequency of low-temperature days and high-temperature days, as detailed in the previous sections, are the primary drivers contributing to increased overwintering mortality rates among A. mellifera colonies across the country.
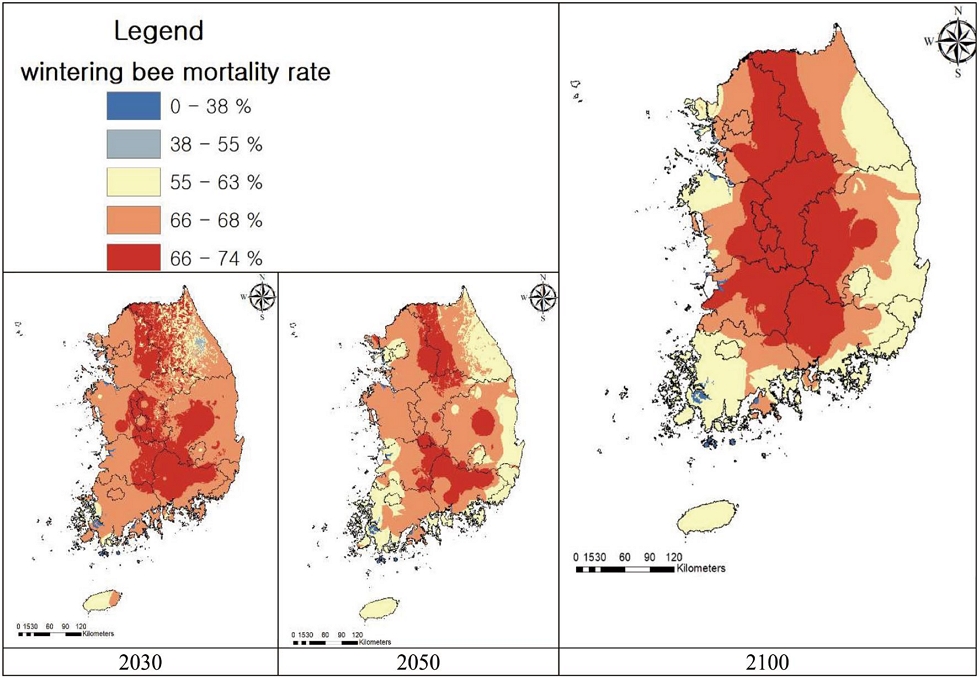
Projected winter mortality rates of A. mellifera colonies in South Korea (2030, 2050, 2100).The figure illustrates the projected rise in winter mortality rates of A. mellifera colonies across South Korea from 2030 to 2100. By 2100, most regions, especially central and southern areas, are expected to experience mortality rates exceeding 66%, driven by warming winters and increased temperature variability, with even northern regions showing substantial increases
In 2030, the mortality rates of A. mellifera colonies are already high in certain regions. Central inland areas like Gyeonggi-do, Chungcheongbuk-do, and Chungcheongnam-do exhibit mortality rates of approximately 66-68%. Similarly, southern coastal regions, including parts of Jeollanam-do and Gyeongsangnam-do, also face high mortality rates due to warming winters and increased temperature variability. In contrast, northern regions, such as Gangwon-do, experience relatively lower mortality rates, with milder increases compared to the central and southern regions. This is likely because their cooler winter temperatures remain more stable, providing better conditions for A. mellifera overwintering.
By 2050, the situation continues to deteriorate. A. mellifera mortality rates rise to over 55% across most regions, with several areas experiencing dramatic increases. Regions such as Daegu, Gyeongsangbuk-do, and Jeollabuk-do stand out as critical hotspots, reporting mortality rates exceeding 68%. In Chungcheong-do, the combination of warming winters and growing temperature variability drives mortality rates to over 63%. Southern coastal areas persist in experiencing high rates, exacerbated by prolonged warm periods and increased stress on A. mellifera colonies. While northern regions, including northern Gangwon-do and northern Gyeonggi-do, maintain relatively lower mortality rates, these areas are also trending upwards, reflecting the widespread impact of climate change.
By 2100, A. mellifera mortality rates will reach alarming levels across South Korea. Most regions, including Gyeonggi-do, Seoul, and Chungcheong-do, are projected to record mortality rates exceeding 66%, with some areas pproaching 74%. Southern regions such as Gyeongsangnam-do and Jeollanam-do are among the most severely affected, with mortality rates surpassing 70%. These high rates are the result of sustained warming winters and reduced energy conservation within A. mellifera colonies, leaving them unable to survive until spring (Truong et al., 2023). Even northern regions, such as northern Gangwon-do, are not immune to these trends, as mortality rates in these areas are projected to rise to 55-63%, demonstrating the pervasive effects of climate change on A. mellifera survival.
A. mellifera are highly sensitive to environmental changes, particularly temperature fluctuations (Kuhlmann et al., 2012). Rising winter temperatures combined with greater day-night temperature variability disrupt the natural dormancy cycle of A. mellifera colonies (Nürnberger et al., 2018). Instead of conserving energy during a cold and stable winter, A. mellifera are forced to remain active, resulting in increased energy expenditure and depleted reserves. These stressors reduce their ability to survive the winter (Seeley and Visscher, 1985). The study predicts that this phenomenon will worsen over time, with winter mortality rates for A. mellifera colonies steadily increasing across South Korea. By 2100, most regions are expected to experience mortality rates exceeding 66%, with southern and central areas facing the most severe challenges.
DISCUSSION
1. Analysis of factors influencing Apis mellifera overwintering mortality
This study provides valuable insights into the climatic factors influencing Apis mellifera overwintering mortality, contributing to a broader understanding of how environmental conditions impact colony health. The regression analysis reveals that low-temperature days during the autumn and winter seasons are the most significant predictors of overwinter losses. The negative impact of autumn low-temperature days suggests that gradual cooling and the formation of the winter cluster are essential for colony survival (Becsi et al., 2021). In contrast, the positive effect of winter low-temperature days highlights the detrimental impact of prolonged cold stress, which forces colonies to expend substantial energy on thermoregulation, depleting their resources and compromising their resilience (Nürnberger et al., 2018).
The negative impact of autumn low-temperature days suggests that gradual cooling and the formation of the winter cluster are essential for colony survival (Becsi et al., 2021). This aligns with previous research indicating that the gradual transition to winter conditions helps prepare colonies for the harsh winter season (Switanek et al., 2016; Calovi et al., 2021). However, low-temperature days during winter combined with high day-night temperature variability can significantly impact A. mellifera overwintering. The combination of warm winter temperatures and large temperature fluctuations disrupts the bees’ natural dormancy cycle, forcing them to remain active and expend more energy to maintain hive temperature. This heightened activity and energy expenditure leave the colonies depleted and less able to survive the winter until spring. This reinforces the importance of temperature regulation as a critical factor in colony survival during winter. The negative correlation between autumn low-temperature days and A. mellifera mortality supports the hypothesis that gradual cooling during autumn helps colonies prepare for winter. Studies have demonstrated that early cooling encourages the formation of winter clusters and promotes energy conservation behaviors, which are essential for overwintering survival (Seeley and Visscher, 1985). Furthermore, research indicates that early autumn cooling stimulates the development of winter bees with physiological adaptations, such as increased fat storage, further enhancing the colony’s ability to withstand long periods of cold (Qin et al., 2019).
The results align with many existing studies, but there are some notable differences. High-temperature days during autumn and winter did not significantly impact A. mellifera mortality in this study. This contrasts with previous research, which found that warm spells, especially in winter, can increase colony activity and deplete resources (Ricigliano et al., 2018). A possible explanation is that the model was constructed using only 2022 data. To accurately predict the impacts of climate change on A. mellifera overwintering mortality, long-term, data-driven research is needed. Regional and environmental variability play a key role, and a comprehensive dataset spanning multiple years would provide a more robust understanding of how temperature fluctuations, both mild and extreme, impact A. mellifera colonies in different climates (Łangowska et al., 2016). Also, autumn precipitation was not a significant predictor of overwintering mortality, differing from earlier studies suggesting wet autumns reduce forage and negatively impact colonies. This discrepancy could be due to localized foraging conditions or beekeeper interventions like artificial feeding (Johannesen et al., 2022).
Regional and environmental variability is a key factor in understanding the complex relationships between climate conditions and A. mellifera behavior and survival (Ricigliano et al., 2018). Climate conditions in different regions can influence how temperature and precipitation affect A. mellifera colonies in unique ways (Łangowska et al., 2016). For instance, studies from North America and Europe have highlighted the negative effects of mild winters on A. mellifera health (Ricigliano et al., 2018), but the conditions in this study’s region may have mitigated the impacts of high-temperature days. Beekeeping practices like artificial feeding and hive insulation could also alleviate environmental stressors and help colonies better withstand temperature variations. Additionally, indoor overwintering may limit A. mellifera colonies’ exposure to external temperature fluctuations (Bordier et al., 2017). Pathogen and parasite interactions can also complicate the relationships between temperature and A. mellifera health (Orčić et al., 2017).
This study emphasizes the importance of low-temperature exposure in autumn and winter for predicting overwintering mortality. Stable autumn cooling helps colonies prepare for the winter season, while prolonged cold spells in winter can deplete colony resources and increase stress (Ricigliano et al., 2018). Beekeepers should ensure their colonies enter the winter season with sufficient food reserves and overall health to mitigate the risks of severe temperature changes, using strategies such as indoor overwintering or hive insulation (Mattila and Otis, 2007). Proactive management of pathogens and parasites, especially for Varroa mites, is also essential to minimize winter losses and support the overall resilience of A. mellifera populations (Ricigliano et al., 2018).
2. Climate change's Impact on Apis mellifera overwintering and ecosystem resilience
These earlier studies have demonstrated that rising temperatures and temperature fluctuations significantly affect the physiology, behavior, and survival of bees, posing major challenges for overwintering survival and ecosystem sustainability (Orčić et al., 2017; Johannesen et al., 2022). For instance, increases in winter temperatures lead to higher energy expenditure in Apis mellifera colonies, which aligns with this study’s findings regarding the heightened energy needs of colonies in warmer overwintering conditions (Ricigliano et al., 2018).
Other research found that increased winter temperatures lead to higher energy expenditure in A. mellifera colonies, which aligns with this study’s findings regarding the heightened energy needs in warmer overwintering conditions (Rajagopalan et al., 2024). Additionally, Lee et al. (2022) investigate how temperature variability affects A. mellifera colony loss during winter, specifically highlighting the influence of unusually warm weather in January and February. These warm spells can disrupt the natural behaviors of bees, potentially leading to higher mortality rates.
This study, however, provides a more nuanced temporal analysis across specific climate change time points and explores the differing impacts across seasonal phases, particularly during the spring-autumn preparation and autumn-winter transition periods. This detailed approach allows for a deeper understanding of how even subtle increases in diurnal temperature range may act as an additional stressor (Stevenson et al., 2015). For example, by 2100, the DTR is projected to increase significantly, especially during preparation periods, potentially exacerbating the physiological demands on bees to regulate internal temperatures within colonies. This builds on findings from global studies that highlight DTR as a critical yet often overlooked factor affecting ecosystem stability (Bordier et al., 2017). While earlier works have focused primarily on general temperature increases (Stabentheiner et al., 2010), this study’s integration of diurnal fluctuations emphasizes the importance of temperature consistency in maintaining pollinator health and colony resilience.
The study also adds to existing knowledge by emphasizing regional variations within Korea. It highlights that southern coastal regions will likely experience the most dramatic temperature changes, which could have severe implications for bee populations localized in these areas. Prior research, including studies from warmer climates such as Queensland, and Australia, shows that similar regional disparities in temperature fluctuations can lead to significant shifts in habitat suitability for bees, underscoring the need for localized adaptation strategies (Kuhlmann et al., 2012). However, the projections of DTR changes in the Korean context specifically underline the need for targeted interventions in regions where DTR increases sharply, rather than a one-size-fits-all national approach (Kim et al., 2023a).
The study also diverges from some prior findings by presenting evidence of how the temperature increases in spring-autumn and autumn-winter preparation periods particularly impact energy management in bees, affecting their ability to stockpile sufficient energy reserves for overwintering. This may be attributed to the Korea’s unique seasonal climate and the rapid rate of projected warming in this study compared to earlier studies focused on temperate or tropical climates, where such marked diurnal temperature increases are less pronounced.
This study builds upon the existing body of research by providing a detailed analysis of the impacts of specific climate change scenarios on A. mellifera populations in Korea. It underscores the necessity for climate adaptation strategies that are both timeand region-specific, addressing temperature increases, diurnal fluctuations, and precipitation changes. These findings highlight the critical importance of temperature stability for the health and survival of overwintering bees.
3. Impact of climate indicators on Apis mellifera overwintering mortality in Korea
This study provides valuable insights into the effects of climate change on bee overwintering mortality in Korea. It focuses on key climate indicators such as average temperature rise, high-temperature days, diurnal temperature range, low-temperature days, and autumn rainfall days. The findings indicate that average temperature rise and high-temperature days are the primary factors affecting overwintering mortality in bees. Consistent with previous research, these factors directly influence energy expenditure and hinder Apis mellifera from accumulating sufficient energy reserves for overwintering (Maes et al., 2021). Higher average temperatures increase energy consumption within colonies, leading to higher mortality rates (Calovi et al., 2021). DTR fluctuations also emerged as a significant factor, as greater temperature variations between day and night increase bees’ energy expenditure, further reducing their overwintering success. This aligns with findings in other studies that link wider DTR to increased metabolic stress in bees, underscoring the need for management interventions in areas with high DTR (Kronenberg and Heller, 1982; Bennett et al., 2023).
Although low-temperature days and autumn rainfall days may be less critical than temperature rise and high-temperature days, they nevertheless play significant roles in overwintering preparation (Bennett et al., 2015). Reduced low-temperature days can hinder A. mellifera ability to adequately prepare for winter, while increased autumn rainfall can interfere with foraging and energy storage activities (Calovi et al., 2021). Using a comprehensive set of climate indices, including temperature rise, high-temperature days, diurnal temperature range, low-temperature days, and autumn rainfall days, provide a more thorough understanding of the factors impacting bee survival compared to relying on a single climate measure (Switanek et al., 2016). These indices allow for the consideration of multiple environmental stressors that collectively influence bee overwintering success, capturing the complexity of real-world climate interactions (Becsi et al., 2021). Collectively, these findings suggest that maintaining optimal temperature ranges and minimizing disruptive weather events during the critical pre-winter months is essential for supporting bee survival.
The study highlights regional variations in bee mortality rates across Korea. In particular, southern coastal and central inland areas experience disproportionately high mortality risks due to pronounced increases in average temperature and high-temperature days. In these regions, the warmer conditions combined with fewer low-temperature days create an environment that makes it exceptionally challenging for bees to adequately prepare for winter (Calovi et al., 2021). In contrast, northern regions and mountainous areas in Gangwon Province tend to have relatively lower mortality rates, as they are less affected by the temperature rises and diurnal temperature range increases. These less impacted areas may therefore serve as important refuges for A. mellifera populations under future climate scenarios (Naeem et al., 2019).
The observed regional differences suggest that the climatic conditions and bee colonies’ adaptability vary significantly across Korea, necessitating a tailored approach to bee conservation. Accordingly, the increased mortality rates in the southern coastal and central inland regions indicate a pressing need for more intensive management practices in these high-risk areas to effectively mitigate climate-induced stress (Kim et al., 2023b).
Our findings align with previous research that highlights the critical roles of temperature rise and high-temperature days in determining overwintering success (Chen et al., 2015; Markle et al., 2020). However, this study extends beyond prior work by examining the impacts of DTR fluctuations and autumn rainfall days, providing a more comprehensive assessment of the various climate stressors on bee ecosystems. These additional factors are particularly relevant in the context of the Korean climate, where seasonal rainfall patterns and temperature fluctuations can significantly influence bee activity and survival. One notable divergence from existing research is our discovery that southern coastal and central inland areas are more severely impacted by increased autumn rainfall days and decreased low-temperature days (Nürnberger et al., 2018). This specificity to the Korean Peninsula reflects unique regional climate characteristics, such as prolonged monsoon seasons and relatively warm autumns (Yi, 2011), which can disrupt bees’ energy storage activities (Kim et al., 2023a). The increased autumn rainfall in these areas, in particular, represents a unique finding that may inform future studies on the interactions between climate change and bee foraging behavior in similar monsoonal climates.
SUMMARY
This study underscores the significant impact of climate change on Apis mellifera overwintering mortality, specifically within the Korean Peninsula. By examining climate change projections for the years 2030, 2050, and 2100, this research provides critical insights into how rising average temperatures, increased diurnal temperature range (DTR), high-temperature days, reduced low-temperature days, and increased autumn precipitation are expected to influence A. mellifera survival.
The study revealed that average temperature rise and high-temperature days have the most profound effects on A. mellifera overwintering mortality. These factors contribute to elevated energy consumption as bees are forced to remain active during warmer winter periods, preventing them from conserving the necessary energy reserves for successful overwintering. Increased DTR, especially in southern and inland regions, further complicates the bees’ thermoregulation, leading to additional energy expenditures that weaken colony resilience.
Moreover, the projected decrease in low-temperature days by 2100, especially in southern coastal and central inland areas, presents a unique challenge for bees. Reduced exposure to cold during the pre-winter period disrupts their ability to transition into the overwintering phase, leaving colonies unprepared for the winter season. The increase in autumn rainfall days also poses a threat by limiting bees’ foraging days, hindering their ability to stockpile adequate resources for winter. These findings suggest that targeted climate adaptation strategies are essential to mitigate the negative effects of climate change on A. mellifera populations. The study recommends that vulnerable regions, particularly the southern coastal and central inland areas, implement region-specific interventions, such as indoor overwintering environments and hive insulation practices, to reduce energy consumption within colonies.
This study has several limitations. It relies heavily on climate projections, which inherently contain uncertainty. The accuracy of the overwintering mortality predictions depends on the reliability and precision of the future climate models used. Additionally, the study focuses on a limited set of climate variables, such as temperature and precipitation, potentially overlooking other important factors that may impact bee survival, including changes in vegetation, pathogen dynamics, and colony-level variations. Furthermore, the model was only validated using 2023 data, and the limited set of variables, such as wind speed, regional microclimates, humidity, pests, diseases, beekeeping practices, and surrounding vegetation, may restrict the model’s ability to accurately predict overwinter mortality. Bee colonies can exhibit diverse responses to environmental stressors, and individual colony characteristics, management practices, and genetic diversity may not be fully captured in the current modeling approach. Additionally, the increased frequency of mite infestations associated with climate change can contribute to the complex dynamics affecting A. mellifera overwintering success.
Overall, this research contributes to a deeper understanding of the relationship between climate change and A. mellifera overwintering mortality. The identified climate indices provide valuable tools for predicting future colony losses and guiding the development of adaptive management strategies. Despite the limitations, the use of climate indices in the model allows for the evaluation of various weather factors and short-term weather anomalies, and it enables the creation of a nationwide predictive model.
To enhance the resilience of A. mellifera populations navigating the challenges of a rapidly changing climate, additional research is necessary to refine predictive models and explore a wider range of factors. These include wind speed, regional microclimates, humidity, the impacts of pests and diseases, variations in beekeeping practices across farms, and the surrounding vegetation. Incorporating these elements will enable the development of more robust and accurate predictive models to guide the implementation of effective adaptive management strategies.
Acknowledgments
This research was supported by the Rural Development Administration research project (PJ014180).
References
-
Abou-Shaara, H. F., A. A. Owayss, Y. Ibrahim and N. K. Basuny. 2017. A review of impacts of temperature and relative humidity on various activities of honeybees. Insectes Soc. 64(4): 455-463.
[https://doi.org/10.1007/s00040-017-0573-8]
-
An, S., G. Park, H. Jung and D. Jang. 2022. Assessment of Future Drought Index Using SSP Scenario in Rep. of Korea. Sustainability14(7): 4252.
[https://doi.org/10.3390/su14074252]
-
Becsi, B., H. Formayer and R. Brodschneider. 2021. A biophysical approach to assess weather impacts on honeybee colony winter mortality. R. Soc. Open Sci. 8(9): 210618.
[https://doi.org/10.1098/rsos.210618]
-
Bennett, M. M., K. M. Cook, J. P. Rinehart, G. D. Yocum, W. P. Kem and K. J. Greenlee. 2015. Exposure to suboptimal temperatures during metamorphosis reveals a critical developmental window in the Solitary Bee, Megachile rotundata. Physiol. Biochem. Zool. 88(5): 508-520.
[https://doi.org/10.1086/682024]
-
Bennett, M. M., K. M. DeBardlabon, J. P. Rinehart, G. D. Yocum and K. J. Greenlee. 2023. Effects of developmental state on low-temperature physiology of the alfalfa leafcutting bee, Megachile rotundata. Bull. Entomol. Res. 113(3): 299-305.
[https://doi.org/10.1017/S0007485321001103]
-
Bordier, C., H. Dechatre, S. Suchail, M. Peruzzi, S. Soubeyrand, M. Pioz, M. Pélissier, D. Crauser, Y. L. Conte and C. Alaux. 2017. Colony adaptive response to simulated heat waves and consequences at the individual level in honeybees (Apis mellifera). Sci. Rep. 7(1): 3760.
[https://doi.org/10.1038/s41598-017-03944-x]
-
Calovi, M., C. M. Grozinger, D. A. Miller and S. C. Goslee. 2021. Summer weather conditions influence winter survival of honeybees (Apis mellifera) in the northeastern United States. Sci. Rep. 11(1): 1553.
[https://doi.org/10.1038/s41598-021-81051-8]
-
Chen, S., S. J. Fleischer, M. C. Saunders and M. B. Thomas. 2015. The Influence of Diurnal Temperature Variation on Degree-Day Accumulation and Insect Life History. PLoS One 10(3): e0120772.
[https://doi.org/10.1371/journal.pone.0120772]
-
Cho, Y., S. Jeong, D. Lee, S. Kim, R. J. Park, L. Gibson, C. Zheng and C. Park. 2021. Foraging trip duration of honeybee increases during a poor air quality episode and the increase persists thereafter. Ecol. Evol. 11(4): 1492-1500.
[https://doi.org/10.1002/ece3.7145]
-
Daly, C., M. D. Halbleib, J. L. Smith, W. Gibson, M. K. Doggett, G. H. Taylor, J. B. Curtis and P. P. Pasteris. 2008. Physiographically sensitive mapping of climatological temperature and precipitation across the conterminous United States. Int. J. Clim. 28(15): 2031-2064.
[https://doi.org/10.1002/joc.1688]
-
Desai, S. D. and R. W. Currie. 2016. Effects of Wintering Environment and Parasite-Pathogen Interactions on Honeybee Colony Loss in North Temperate Regions. PLoS One 11(7): e0159615.
[https://doi.org/10.1371/journal.pone.0159615]
-
Dunne, J. P., J. G. John, E. Shevliakova, R. J. Stouffer, J. P. Krasting, S. Malyshev, P. D. D. Milly, L. T. Sentman, A. Adcroft, W. Cooke, K. A. Dunne, S. M. Griffies, R. Hallberg, M. Harrison, H. Levy, A. T. Wittenberg, P. J. Phillips and N. Zadeh. 2013. GFDL’s ESM2 Global Coupled Climate-Carbon earth system models. Part II: carbon system formulation and baseline simulation characteristics. J. Clim. 26(7): 6646-6665.
[https://doi.org/10.1175/JCLI-D-12-00150.1]
-
Harrison, J. F. and J. H. Fewell. 2002. Environmental and genetic influences on flight metabolic rate in the honeybee, Apis mellifera. Comp. Biochem. Physiol. A Mol. Integr. Physiol. 133(2): 323-233.
[https://doi.org/10.1016/S1095-6433(02)00163-0]
-
Heinrich, B. 1996. How the honeybee regulates its body temperature. Bee World 77(3): 130-137.
[https://doi.org/10.1080/0005772X.1996.11099304]
-
Jang, K., M. S. Won and S. H. Yoon. 2017. Evaluation of the satellite-based air temperature for all sky conditions using the automated mountain meteorology station (AMOS) records: Gangwon province case study. Korean. J. Agric. For. Meteorol. 19(1): 19-26.
[https://doi.org/10.5532/KJAFM.2017.19.1.19]
-
Johannesen, J., S. Wöhl, S. Berg and C. Otten. 2022. Annual fluctuations in winter colony losses of Apis mellifera L. are predicted by honey flow dynamics of the preceding year. Insects 13(9): 829.
[https://doi.org/10.3390/insects13090829]
-
Kim, H.-R., M. Moon, J. Yun and K. Ha. 2023a. Trends and spatio-temporal variability of ssummer mean and extreme precipitation across South Korea for 1973-2022. Asia-Pac. J. Atmos. Sci. 59: 385-398.
[https://doi.org/10.1007/s13143-023-00323-7]
-
Kim, J. P. 2014. Estimation of high-resolution daily precipitation using a modified PRISM model. KSCE J. Civ. Eng. 34(4): 1139-1150.
[https://doi.org/10.12652/Ksce.2014.34.4.1139]
-
Kim, M., S. Bak and C. Jung. 2023b. Modeling abundance and risk impact of Vespa velutina nigrithorax (Hymenoptera: Vespidae). Sci. Rep. 13(1): 13616.
[https://doi.org/10.1038/s41598-023-40016-9]
-
Kim, Y., J. Kim, Y. Oh, S. Lee, S. Song, E. Joung, S. Lee, S. J. Lee and B. Moon. 2016. Prevalence of honeybee (Apis mellifera) disease in Daejeon. Korean. J. Vet. Serv. 39(4): 253-258.
[https://doi.org/10.7853/kjvs.2016.39.4.253]
-
Kronenberg, F. and H. C. Heller. 1982. Colonial thermoregulation in honeybees (Apis mellifera). J. Comp. Physiol. B 148(1): 65-76.
[https://doi.org/10.1007/BF00688889]
-
Kuhlmann, M., D. Guo, R. Veldtman and J. S. Donaldson. 2012. Consequences of warming up a hotspot: species range shifts within a centre of bee diversity. Divers. Distrib. 18(9): 885-897.
[https://doi.org/10.1111/j.1472-4642.2011.00877.x]
-
Kulhanek, K., N. Steinhauer, K. Rennich, D. M. Caron, R. R. Sagili, J. Pettis, J. Ellis, M. Wilson, J. Wilkes, D. R. Tarpy, R. Rose, K. Lee, J. Rangel and D. vanEngelsdorp. 2017. A national survey of managed honeybee 2015-2016 annual colony losses in the USA. J. Apic. Res. 56(4): 328-340.
[https://doi.org/10.1080/00218839.2017.1344496]
-
Łangowska, A., M. Zawilak, T. H. Sparks, A. Głazaczow, P. W. Tomkins and P. Tryjanowski. 2016. Long-term effect of temperature on honey yield and honeybee phenology. Int. J. Biometeorol. 61(6): 1125-1132.
[https://doi.org/10.1007/s00484-016-1293-x]
-
Lee, S. J., S. H. Kim, J. Y. Lee, J. H. Kang, S. M. Lee, H. J. Park, J. C. Nam and C. Jung. 2022. Impact of ambient temperature variability on the overwintering failure of honeybees in South Korea. J. Apic. 37(3): 331-347.
[https://doi.org/10.17519/apiculture.2022.09.37.3.331]
-
Li, X., W. Ma, J. Shen, D. Long, Y. Feng, W. Su, K. Xu, Y. Du and Y. Jiang. 2019. Tolerance and response of two honeybee species Apis cerana and Apis mellifera to high temperature and relative humidity. PLoS One 14(6): e0217921.
[https://doi.org/10.1371/journal.pone.0217921]
-
Lim, C. H., S. Yoo, Y. Choi, S. W. Jeon, Y. Son and W. Lee. 2018. Assessing climate change impact on forest habitat suitability and diversity in the Korean peninsula. Forests 9(5): 259-265.
[https://doi.org/10.3390/f9050259]
-
Maes, P., A. Floyd, B. M. Mott and K. E. Anderson. 2021. Overwintering honeybee colonies: effect of worker age and climate on the hindgut microbiota. Insects 12(3): 224.
[https://doi.org/10.3390/insects12030224]
-
Mardan, M. and P. G. Kevan. 2002. Critical temperatures for survival of brood and adult workers of the giant honeybee, Apis dorsata (Hymenoptera: Apidae). Apidologie 3(3): 295-301.
[https://doi.org/10.1051/apido:2002017]
-
Markle, C. E., P. Moore and J. M. Waddington 2020. Temporal variability of overwintering conditions for a species-at-risk snake: Implications for climate change and habitat management. Glob. Ecol. Conserv. 22: e00923.
[https://doi.org/10.1016/j.gecco.2020.e00923]
-
Mattila, H. R. and G. W. Otis. 2007. Dwindling pollen resources trigger the transition to bloodless populations of long-lived honeybees each autumn. Ecol. Entomol. 32(5): 496-505.
[https://doi.org/10.1111/j.1365-2311.2007.00904.x]
-
McAfee, A., A. Chapman, H. Higo, R. M. Underwood, J. Milone, L. J. Foster, M. M. Guarna, D. R. Tarpy and J. S. Pettis. 2020. Vulnerability of honeybee queens to heat-induced loss of fertertility. Nat. Sustain. 3(5): 367-376.
[https://doi.org/10.1038/s41893-020-0493-x]
-
Millard, J., C. L. Outhwaite, S. Ceauşu, L. G. Carvalheiro, F. D. Silva, L. V. Dicks, J. Ollerton and T. Newbold. 2023. Key tropical crops at risk from pollinator loss due to climate change and land use. Sci. Adv. 9(41): eadh0756.
[https://doi.org/10.1126/sciadv.adh0756]
-
Minder, J. R., P. W. Mote and J. D. Lundquist. 2010. Surface temperature lapse rates over complex terrain: Lessons from the cascade mountains. J. Geophys. Res. Atm. 115: D14122.
[https://doi.org/10.1029/2009JD013493]
-
Naeem, M., M. Liu, J. Huang, G. Ding, G. S. Potapov, C. Jung and J. An. 2019. Vulnerability of East Asian bumblebee species to future climate and land cover changes. Agric. Ecosyst. Environ. 277: 11-20.
[https://doi.org/10.1016/j.agee.2019.03.002]
-
Nürnberger, F., S. Härtel and L. Steffan-Dewenter. 2018. The influence of temperature and photoperiod on the timing of brood onset in hibernating honeybee colonies. PeerJ 6: e4801.
[https://doi.org/10.7717/peerj.4801]
-
Orčić, S., T. V. Nikolić, J. Purać, B. Šikoparija, D. Blagojević, E. Vukašinović, N. Plavša, J. Stevanović and D. Kojić. 2017. Seasonal variation in the activity of selected antioxidant enzymes and malondialdehyde level in worker honeybees. Entomol. Exp. Appl. 165(2-3): 120-128.
[https://doi.org/10.1111/eea.12633]
-
Overturf, K. A., N. Steinhauer, R. Molinari, M. Wilson, A. C. Watt, R. M. Cross, D. vanEngelsdorp, G. R. Williams and S. R. Rogers. 2022. Winter weather predicts honeybee colony loss at the national scale. Ecol. Ind. 145: 109709.
[https://doi.org/10.1016/j.ecolind.2022.109709]
-
Owen, E. L., J. S. Bale and S. A. L. Hayward. 2013. Can winter-active bumblebees survive the cold? Assessing the cold tolerance of Bombus terrestris audax and the effects of pollen feeding. PLoS One 8(11): e80061.
[https://doi.org/10.1371/journal.pone.0080061]
-
Özelkan, E., S. Bagis, B. B. Üstündağ, E. C. Özelkan, M. Yücel and C. Örmeci. 2013. Land surface temperature-based spatial interpolation using a modified Inverse Distance Weighting method. Second International Conference on Agro-Geoinformatics (Agro-Geoinformatics), Fairfax, VA, USA, 2013, pp. 110-115.
[https://doi.org/10.1109/Argo-Geoinformatics.2013.6621890]
-
Park, S., S. K. Park, J. W. Lee and Y. Park. 2018. Geostatistical assessment of warm-season precipitation observations in Korea based on the composite precipitation and satellite water vapor data. Hydrol. Earth Syst. Sci. 22(6): 3435-3452.
[https://doi.org/10.5194/hess-22-3435-2018]
-
Parson, E. A. and D. M. A. Karwat. 2011. Sequential climate change policy. Wiley Interdiscip. Rev. Clim. 2(5): 744-756.
[https://doi.org/10.1002/wcc.128]
-
Qin, M., H. Wang, Z. Liu, Y., Wang, W. Zhang and B. Xu. 2019. Changes in cold tolerance during the overwintering period in Apis mellifera ligustica. J. Apic. Res. 58(5): 702-709.
[https://doi.org/10.1080/00218839.2019.1634461]
-
Quinlan, G. M., R. Isaacs, C. R. V. Otto, A. H. Smart and M. O. Milbrath. 2023. Association of excessive precipitation and agricultural land use with honeybee colony performance. Landsc. Ecol. 38(6): 1555-1569.
[https://doi.org/10.1007/s10980-023-01638-6]
-
Rajagopalan, K., G. DeGrandi-Hoffman, M. J. Pruett, V. P. Jones, V. Corby-Harris, J. Pireaud, R. Curry, B. K. Hopkins and T. D. Northfield. 2024. Warmer autumns and winters could reduce honeybee overwintering survival with potential risks for pollination services. Sci. Rep. 14 (1): 5410.
[https://doi.org/10.1038/s41598-024-55327-8]
-
Randerson, J. T., K. Lindsay, E. Munoz, W. Fu, J. K. Moore, F. M. Hoffman, N. M. Mahowald and S. C. Doney. 2015. Multi-century changes in ocean and land contributions to the climate-carbon feedback. Global Biogeochem. Cycles 29(6): 744-759.
[https://doi.org/10.1002/2014GB005079]
-
Ricigliano, V., B. M. Mott, A. Floyd, D. Copeland, M. J. Carroll and K. E. Anderson. 2018. Honeybees overwintering in a southern climate: longitudinal effects of nutrition and queen age on colony-level molecular physiology and performance. Sci. Rep. 8(1): 10475.
[https://doi.org/10.1038/s41598-018-28732-z]
-
Salehizadeh, A., M. Khodagholi and A. Gandomkar. 2020. Temperature conditions for determination of beekeeping regions in the light of climate change. Case study: Fars Province. Environ. Clim. Technol. 24(1): 88-104.
[https://doi.org/10.2478/rtuect-2020-0006]
-
Schenk, M., O. Mitesser, T. Hovestadt and A. Holzschuh. 2018. Overwintering temperature and body condition shift emergence dates of spring-emerging solitary bees. PeerJ 6: e4721.
[https://doi.org/10.7717/peerj.4721]
-
Seeley, T. D. and P. K. Visscher. 1985. Survival of honeybees in cold climates: the critical timing of colony growth and reproduction. Ecol. Entomol. 10(1): 81-88.
[https://doi.org/10.1111/j.1365-2311.1985.tb00537.x]
-
Sgolastra, F., W. P. Kemp, J. S. Buckner, T. L. Pitts-Singer, S. Maini and J. Bosch. 2011. The long summer: Pre-wintering temperatures affect metabolic expenditure and winter survival in a solitary bee. J. Insect Physiol. 57(12): 1651-1659.
[https://doi.org/10.1016/j.jinsphys.2011.08.017]
-
Siegel, D. A., T. DeVries, I. Cetinić and K. Bisson. 2022. Quantifying the ocean’s biological pump and its carbon cycle impacts on global scales. Ann. Rev. Mar. Sci. 15(1): 329-356.
[https://doi.org/10.1146/annurev-marine-040722-115226]
-
Sinclair, B. J. 2014. Linking energetics and overwintering in temperate insects. J. Therm. Biol. 54(5): 5-11.
[https://doi.org/10.1016/j.jtherbio.2014.07.007]
-
Stabentheiner, A. and H. Kovac. 2014. Energetic optimization of foraging honeybees: flexible change of strategies in response to environmental challenges. PLoS One 9(8): e105432.
[https://doi.org/10.1371/journal.pone.0105432]
-
Stabentheiner, A., H. Kovac and R. Brodschneider. 2010. Honeybee Colony Thermoregulation - Regulatory Mechanisms and Contribution of Individuals in Dependence on Age, Location and Thermal Stress. PLoS One 5(1): e8967.
[https://doi.org/10.1371/journal.pone.0008967]
-
Steinhauer, N., K. Rennich, M. Wilson, D. M. Caron, E. J. Lengerich, J. Pettis, R. Rose, J. A. Skinner, D. R. Tarpy, J. Wilkes and D. vanEngelsdorp. 2014. A national survey of managed honey bee 2012-2013 annual colony losses in the USA: results from the Bee Informed Partnership. J. Apic. Res. 53(1): 429-443.
[https://doi.org/10.3896/IBRA.1.53.1.01]
-
Stevenson, T. J., M. E. Visser, W. Arnold, P. Barrett, S. M. Biello, A. Dawson, D. L. Denlinger, D. M. Dominoni, F. J. P. Ebling, S. Elton, N. P. Evans, H. M. Ferguson, R. G. Foster, M. Hau, D. T. Haydon, D. G. Hazlerigg, P. D. Heideman, J. G. C. Hopcraft, N. N. Jonsson, V. Kumar, G. A. Lincoin, R. MacLeod, S. A. M. Martin, M. Martinez-Bakker, R. J. Nelson, T. Reed, J. E. Robinson, D. Rock, W. J. Schwartz, I. Steffan-Dewenter, E. Tauber, S. J. Thackeray, C. Umstatter, T. Yoshimura and B. Helm. 2015. Disrupted seasonal biology impacts health, food security and ecosystems. Proc. Biol. Sci. 282(1817): 20151453.
[https://doi.org/10.1098/rspb.2015.1453]
-
Switanek, M. B., K. Crailsheim, H. Truhetz and R. Brodschneider. 2016. Modelling seasonal effects of temperature and precipitation on honeybee winter mortality in a temperate climate. Sci. Total Environ. 579: 1581-1587.
[https://doi.org/10.1016/j.scitotenv.2016.11.178]
-
Truong, A., M. S. Yoo, S. K. Seo, T. J. Hwang, S. S. Yoon and Y. S. Cho. 2023. Prevalence of honeybee pathogens and parasites in South Korea: A five-year surveillance study from 2017 to 2021. Heliyon 9(2): e13494.
[https://doi.org/10.1016/j.heliyon.2023.e13494]
-
vanEngelsdorp, D., J. Hayes, R. M. Underwood and J. S. Pettis. 2008. A survey of honey bee colony losses in the U.S., Fall 2007 to Spring 2008. PLoS One 3(12): e4071.
[https://doi.org/10.1371/journal.pone.0004071]
-
Yan, Y., T. Onishi and K. Hiramatsu. 2015. Impacts of different spatial temperature interpolation methods on snowmelt simulations. Hydrol. Res. Lett. 9(2): 27-34.
[https://doi.org/10.3178/hrl.9.27]
-
Yi, S. 2011. Holocene Vegetation Responses to East Asian Monsoonal Changes in South Korea. Climate Change - Geophysical Foundations and Ecological Effects. pp. 157-165.
[https://doi.org/10.5772/23920]