
Development of Ultra-Rapid Reverse Transcription Real-Time PCR for Detection against Black Queen Cell Virus in Honeybee
Abstract
Black Queen Cell Virus (BQCV) is one of the most prevalent viruses in honeybee, and could not be recognized by any infected symptoms in the adult bees. Thus, a newly developed method for rapid RNA isolation and cDNA generation from honeybee samples will be useful and easy to evaluate the presence of BQCV. A significant improvement by only one-step RNA isolation for 10 minutes made possible to detect BQCV RNAs at high quantity. cDNAs were also generated directly from isolated BQCV RNAs by different primer sets for 1 minutes and were subsequently applied to Ultra-Rapid Real-Time PCR by using microchip of 6μl reaction volume with extremely short time in each step of PCR. This system provided the ultra-high speed reaction (30 cycles in less than 10 min) including melting temperature analysis for amplified BQCV products. These results suggest that BQCV detection can be completed within 6 min 42 sec, and the Ultra-Rapid Real-time PCR is sensitive, reliable and time-saving for monitoring BQCV in honeybee.
Keywords:
Black queen cell virus (BQCV), Honeybee, Ultra-Rapid Real-Time PCRINTRODUCTION
Black queen cell virus (BQCV) is one of the prevalent viruses of honeybee, causes the death of queen larvae and pupae. And the name of this virus is derived from the dark colour areas on the wall of cells containing infected pupae as typical symptoms (Bailey and Woods, 1977). The infection of BQCV in queen cell has been reported in Australia (Anderson, 1993). BQCV is a single-stranded Picorna-like virus and constructs of 8,550 nucleotides with two open reading frames (ORFs): a 5’-proximal ORF (a putative replicase) and a 3’-proximal ORF (a capsid protein) (Leat et al., 2000). However, BQCV is found out in the co-infection with a Microsporidian parasite, Nosema apis (Allen and Ball, 1996; Bailey et al., 1983) and indicated that the mortality of bees infested with this parasite. More controversial issue is the relationship between BQCV and the Varroa destructor mite (Tentcheva et al., 2004; Chatawannakul et al., 2006) suggesting that this virus and mites affect together to bee colonies. The term “bee parasitic mite syndrome” is used to describe a disease complex of bee colonies with mites and viruses infestation. Consequently, a high mortality of bees is accompanied (Shimanuki et al., 1994).
For many years, the classical diagnostic methods for the detection of honeybee viruses based on electron microscopy (EM) and agar gel immunodiffusion (AGID) test have been mainly used for the detection of honeybee viruses (Allen and Ball, 1996). However, these methods still have met weak points; time-consuming, low specificity and sensitivity. Since quantitative Real-Time PCR assay (qRT-PCR) has been developed, several studies have been using this assay for the detection of honeybee viruses (Bakonyi et al., 2002; Benjeddou et al., 2001; Blanchard et al., 2007; Topley et al., 2005) and the application of RTPCR for simultaneous detection of different viruses in a sample has reduced significantly time, offered cost-saving advantages in case of large numbers of samples are analyzed. Recently, the chip-based thermal cycle has been developed to focusing on the improvement of low speed and temperature instability inside the reaction tubes (Kricka and Wilding, 2003; Mello, 2001). Nowadays, a novel device, chip-based real-time PCR thermal cycle GenSpector TMC-1000 (Samsung, Suwon, Korea) is produced to gathering advantages of micro-PCR as well as of Real-Time PCR (Cho et al., 2006; Huh et al., 2006). Several scientists have detected successfully honeybee viruses by using Ultra-Rapid Real-Time PCR (URRTPCR) and confirmed constantly this diagnostic assay is rapid, reliable, sensitive and specific (Kim et al., 2007; Lee et al., 2007). This PCR system can complete 40 thermal cycles for less than 20 min (Cho et al., 2006).
In this study, we describe the development and evaluation of new protocols for RNA isolation from honeybee samples and cDNA synthesis. To date, most of viral detection studies over the world have been focusing on viral detection methods without having any time-concerning of RNA isolation and cDNA generation. Our result should become the first scientific recognition by shortening approximately for 10 min in RNA isolation and 1 min in cDNA synthesis. BQCV is able to detect directly from cDNA for 5 min 42 sec by applying to Ultra-Rapid Real-Time PCR detection. Finally, the total detection time was reduced remarkably in the whole process, making easily and time-saving for monitoring BQCV in field. Moreover, this newly advantageous method will be a valuable suggestion for further viral detection assays not only honeybee viruses but also other viral diseases even in human.
MATERIALS AND METHODS
Collection of honeybee samples
Samples consisted of only adult bees were directly taken from the bee hive frames by the beekeepers in Kyonggi University, Korea and sent to the laboratory facilities under specific instructions to maintain them frozen until processed in the laboratory. Then, samples of BQCV-infected honeybees were selected by a simply PCR detection.
10 min in RNA isolation (Modified Takara protocol)
2 adult bees were crushed by cleaned mortars and pestles and total RNAs were extracted by using 1ml of RNAiso Plus (Total RNA extraction reagent) (Takara, Japan). Powdery honeybees were collected to 1.5ml Eppendorf tubes and then 1ml of RNAiso Plus was supplemented to each tube. To separating parts of extraction, 200μl of chloroform was immediately added. After this step, the general process was shortened by only one-step centrifugation of cell lysate at 13,000 rpm for 4 min at 4°C. Then, total RNAs were collected from the upper phase and can be directly used for cDNA generation. The collected volume of solution contained RNAs was 400μl and obtained a very high yield of the viral RNAs. The total RNAs concentration was determined by Biophotometer (Eppendorf, Germany) and maintained at -20°C (Fig. 1).
Modified the incubation time of M-MLV Reverse Transcriptase (Bioneer, Korea) in case of using different primer sets
The extracted RNAs were reverse transcribed by MMLV Reverse Transcriptase (Bioneer, Korea). Briefly, 2μg of total RNAs were incubated with 100 pmol of oligo d(T) 18 (Bionics, Korea) or specific reverse primer sets for BQCV detection (BQCV-VP1-R2 and BQCV-VP3-R1) at 65°C for 10 min, and then the cDNA synthesis reactions were performed at 42°C for 60, 30, 15 5, 1 min respectively and each reaction was inactivated at 95°C for 5 min. Subsequently, cDNAs were ready for Real-Time PCR or Ultra-Rapid Real-Time PCR detection. Afterward, we performed the time minimization of RNA denaturation and Reverse Transcriptase inactivation steps, the time was set as 10, 5, 3, 1, 0 min for each one and the temperature were 65°C and 95°C, respectively.
Specific primers for cDNA synthesis and Ultrarapid real-time PCR
Both two pairs of primers were designed to amplify conserved regions of BQCV genome. The structural protein genes which were identified by a previous study on analysis of the complete genome of BQCV (Leat et al., 2000) were selected. Our designs based on different sequences published previously in the GenBank database (accession numbers AF183905.1 and KR074231) and using an Oligonucleotide Properties Calculator software to evaluate (Table 1). Both sets of primers were chosen according to their annealing temperatures as they could share the same thermo cycler program. Of these primer sets, BQCV-VP1-R2 and BQCV-VP3-R1 were used for cDNA generation. The primers were synthesized by Bionics Corporation (Bionics, Korea).
Preparation of DNA templates (pBX-BQCV-VP1 and pGEM-BQCV-VP3)
PCR amplicons were amplified from structural genes of BQCV by using specific pairs of primer named BQCVVP1-F2; BQCV-VP1-R2 and BQCV-VP3-F1; BQCVVP3-R1 (Table 1). The obtained PCR products were inserted to pBlueXcm vector (GenClone, Korea) and pGEM-3Zf(+) vector (Promega, USA) and should be used as positive controls for detection of BQCV by Real-Time PCR or URRT-PCR detection. The recombinant DNAs named as pBX-BQCV-VP1 and pGEM-BQCV-VP3 were extracted using DNA-spinTM Plasmid DNA Purification Kit (iNtRON Biotechnology, Korea) following tightly to the manufacturer’s instructions. Plasmid DNAs concentration was determined using Biophotometer (Eppendorf, Germany) and stored at -20°C.
Quantitative Real-Time PCR (qRT-PCR) and Ultra-Rapid Real-Time PCR (URRT-PCR) amplification
Real-Time PCR assay was developed based on SYBR green detection. All reactions were carried out with ExicyclerTM Quantitative Thermal Block (Bioneer, Korea). The procedure was performed in a final volume of 20μl containing HiPi Real-Time PCR 2x Master Mix (SYBR green) (Elpisbio, Korea), 10 pmol of each primer and templates DNA or cDNA. We evaluated the best annealing temperatures for both of primer sets by a gradient qRTPCR. In URRT-PCR, all reactions were conducted on a GenSpector® TMC-1000 instrument (Samsung, Korea) with microchips and SYBR green-containing premix. The total reaction volume was 6μl containing 2x SsoFastTM EvaGreen® Supermix (Bio-rad, USA), primers (10 pmol each) and templates DNA or cDNA. Standard reaction conditions were followed one pre-denaturation beginning step at 95°C for 30 sec and then 40 cycles of denaturation at 95°C for 5 sec, annealing and extension at 60°C for 5 sec. The heating and cooling rate was 0.1°C/sec for all steps. The melting temperature analysis was performed after amplification.
RESULTS AND DISCUSSION
10 min for RNA isolation and a confirmation by Real-Time PCR using cDNA
The standard protocol for RNA isolation (Takara, Japan) normally required to completing within 60 min. Instead of a long process might affect to RNA quality and yield due to RNAse contamination, the time for RNA isolation step was reduced to 10 min by one-step centrifugation of cell lysate at 13,000 rpm, 4°C. Following the phase separation, RNA was collected from the upper phase and used 2μg for cDNA generation. cDNA was synthesized under a common condition using 100 pmol of primer oligo d(T) 18 and followed an instruction of M-MLV Reverse Transcriptase (Bioneer, Korea). Subsequently, a qRT-PCR was performed using cDNA. PCR products were successfully amplified using two pairs of primer sets: β-actin-F/β-actin-R and BQCV-VP3-F1/BQCV-VP3-R1. The results were described clearly in Fig. 2.
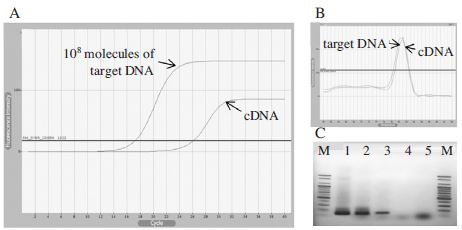
Detection limit of BQCV using cDNA synthesized from RNA isolated for 10 min. Panel A showed fluorescence curves of qRT-PCR using BQCV-VP3-F1/BQCV-VP3-R1 primer set. The result was corresponding to the amplification from 108 molecules of a recombinant pGEM-BQCV-VP3 and cDNA. Panel B showed the melting analysis results of the same PCR products in the range of 60~94°C. In panel C, all PCR products were identified on an agarose gel with the correct sizes of BQCV-VP3 (131bp) and β-actin gene (151bp). Lane 1 and 2 were PCR products amplified from recombinant clone pGEM-BQCV-VP3 and cDNA, respectively. Lane 3 was β-actin gene amplification from cDNA. Lane 4 and 5 were negative controls for BQCV-VP3 and β-actin genes, respectively (PCR without template DNA). As the result, 105 of target DNA molecules were amplified from initial cDNA template.
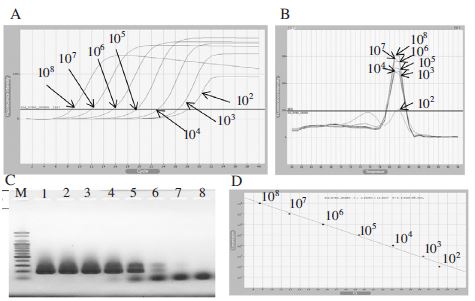
Detection limit of initial template DNA using BQCVVP1-F2/BQCV-VP1-R2 primer set. Panel A showed fluorescence curves of qRT-PCR in a serial dilution to 102 DNA molecules. Panel B showed the melting analysis results of the same PCR products in the range of 60~94°C. Panel C was PCR products were loaded on 1.5% agarose gel, PCR products of 176bp existed in 6 lanes. Lane 1 to 7 were corresponding to a serial dilution of template DNA from 108 to 102 DNA molecules. Lane 8 was a negative control (without DNA template). Panel D was the result of regression analysis of PCR products. The linear relationship between quantities of initial template and Ct values was fairly accepted. In this case, 103 DNA molecules seemed to be a detection limit.
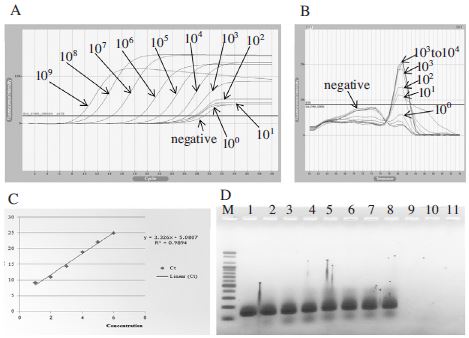
Detection limit of initial template DNA using BQCVVP3-F1/BQCV-VP3-R2 primer set. Panel A showed fluorescence curves of qRT-PCR in a serial dilution to 10o DNA molecules. Panel B showed the melting analysis results of the same PCR products in the range of 60~94°C. Panel C was the result of regression analysis of PCR products. The linear relationship between quantities of initial template and Ct values was fairly accepted. Panel D was PCR products were loaded on 1.5% agarose gel, PCR products of 131 bp existed in 8 lanes. Lane 1 to 10 were corresponding to a serial dilution of template from 109 to 10o DNA molecules. Lane 11 is a negative control (PCR product without DNA template). In this case, 102 DNA molecules was a detection limit.
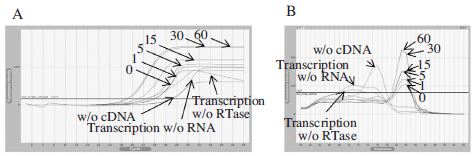
Real-Time PCR using cDNA generated by oligo d(T) 18. Panel A showed fluorescence curves of qRT-PCR in a time reduction of cDNA synthesis at 42°C from 60 to under 1 min and 3 different negative controls were used for making the experimental accuracy. Panel B showed the melting analysis results of the same PCR products in the range of 60~94°C. From this result, we can generate cDNA for 1 min using oligo d(T) 18.
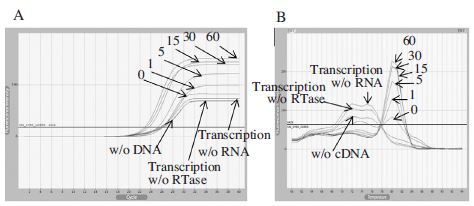
Real-Time PCR using cDNA generated by BQCV-VP3-R1. Panel A showed fluorescence curves of qRT-PCR in a time reduction of cDNA synthesis at 42°C from 60 to under 1 min and 3 different negative controls were used for the experimental accuracy. Panel B showed the melting analysis results of the same PCR products in the range of 60~94°C. From this result, we can confirm that 1 min for cDNA synthesis using this primer is available for detect BQCV by Real-Time PCR.
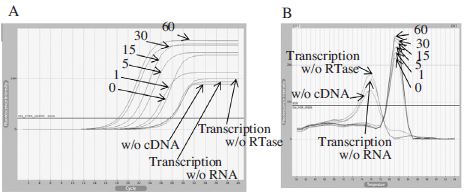
Real-Time PCR using cDNA generated by BQCV-VP1-R2. Panel A showed fluorescence curves of qRT-PCR in a time reduction of cDNA synthesis at 42°C from 60 to under 1 min and 3 different negative controls were used for the experimental accuracy. Panel B showed the melting analysis results of the same PCR products in the range of 60~94°C. This primer can also generate cDNA for 1 min and it showed the higher fluorescence intensity than oligo d(T) 18 or BQCV-VP3-R1.
Evaluation of primer selection by Real-Time PCR
To determine the sensitivity of primer sets, two pairs of primers (Table 1) were used for qPCR amplification. The first primer set named as BQCV-VP1-F2/BQCV-VP1-R2 was designed based on the genome sequence (GenBank Accession No. AF183905.1) and amplified a 176 bp PCR product specific to the target gene. The second primer set named BQCV-VP3-F1/BQCV-VP3-R1 was designed based on the genome sequence (GenBank Accession No. KR074231) and amplified a 131 bp PCR product. The DNA templates were serially diluted 10-fold with no contamination and 1μl of each one was applied to qPCR detection. As the result, BQCV-VP1-F2/BQCV-VP1-R2 can detect BQCV until 103 DNA molecules (Fig. 3) and the detection limit using BQCV-VP3-F1/BQCV-VP3-R1 was 102 DNA molecules (Fig. 4).
The assessment of cDNA synthesis using oligo d(T) 18
The incubation time for M-MLV Reverse Transcriptase (Bioneer, Korea) was gradually reduced from 60 min to less than 1 min. M-MLV Reverse Transcriptase is an enzyme which maintains its activity at room temperature (37°C) and 42°C. Thus, in our study we examined this enzyme activity at 42°C and at room temperature less than 1 min performance. The Real-Time PCR result presented in Fig. 5 pointed out BQCV cDNA is able to be generated in all conditions even at room temperature for a few sec. However, in this case, the fluorescent intensity seemed to be low. Thus, we suggest that the experimental condition at 42°C for 1 min is reasonable for cDNA synthesis using oligo d(T) 18.
The assessment of cDNA synthesis using BQCV-VP3-R1
Black queen cell virus is a positive single-stranded RNA. Therefore, a reverse primer might be useful and obtain a higher specificity for cDNA synthesis than a primer oligo d(T) 18. To clear this point, we designed a reverse primer BQCV-VP3-R1 based on the published nucleotide sequence (GeneBank Accession No. KR074231). The activity of M-MLV Reverse Transcriptase was evaluated in case of an incubation time reduction. A qRT-PCR was subsequently performed and showed that 1 min for cDNA synthesis using this primer is available for detecting BQCV by Real-Time PCR (Fig. 6). All PCR products matched the same melting temperature.
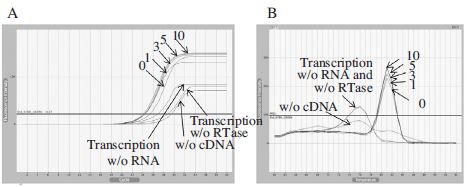
Real-Time PCR using cDNA generated by BQCV-VP1-R2 primer for different RNA denaturation time. Panel A showed fluorescence curves of qRT-PCR in a time reduction of RNA denaturation time at 65°C from 10 to under 1 min and 3 different negative controls were used for the experimental accuracy. Panel B showed the melting analysis results of the same PCR products in the range of 60~94°C. From this result, we can combine RNA denaturation and cDNA synthesis to one step.
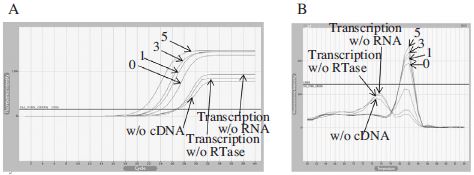
Real-Time PCR using cDNA generated by BQCV-VP1-R2 primer for different RTase inactivated time. Panel A showed fluorescence curves of qRT-PCR in a time reduction of RTase inactivated time at 95°C from 5 to under 1 min and 3 different negative controls were used for the experimental accuracy. Panel B showed the melting analysis results of the same PCR products in the range of 60~94°C. From this result, we can conclude that RTase inactivation step could not affect much to the result of qRT-PCR.
The assessment of cDNA synthesis using BQCV-VP1-R2
To compare the efficiency between specific reverse primers for cDNA synthesis, another structural gene sequence named BQCV-VP1 was selected to design a reverse primer. This primer was examined the specificity using Primer Blast (NCBI) and based on the published nucleotide sequence (GeneBank Accession No. AF18390-5.1). Following the same method for primer assessment, PCR products from cDNAs in Fig. 7 proved that this primer can work well to cDNA synthesis. A high fluorescence intensity even 1 min condition was observed in a melting temperature analysis demonstrated that this primer was the best choice for cDNA generation.
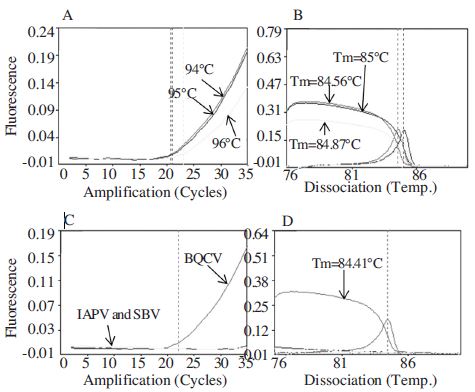
Minimal detection time using BQCV cDNA in URRTPCR and specificity of detection primer. Panel A showed fluorescence curves of URRT-PCR during the amplification period. Panel B showed the melting analysis results of the same PCR products in the range of 76~90°C. Panel C showed fluorescence curves of URRT-PCR in the amplification of 3 different cDNAs from BQCV, IAPV and SBV. Panel D showed the melting analysis results of the same PCR products in the range of 60~94°C. Only BQCV can be detected in this results.
Minimize time for RNA denaturation and Reverse Transcription inactivation steps
For cDNA synthesis using M-MLV Reverse Transcriptase (Bioneer, Korea), there are three different steps: RNA denaturation, M-MLV Reverse Transcriptase activity and Reverse Transcription inactivation. After confirm that 1 min is enough for M-MLV Reverse Transcriptase activity by using BQCV-VP1-R2 primer, we preferred to minimize time for two remaining steps. We recognized that RNA could be denatured at 42°C in a combination with MMLV Reverse Transcriptase activity step. It meant that a general RNA denaturation step at 65°C for 10 min should be eliminated (Fig. 8). During the time reduction of Reverse Transcription inactivation step, BQCV can be amplified in all conditions. Moreover, RTase inactivation step seemed to be not necessary because a good amplification from cDNA without RTase inactivation step was clearly observed in Fig. 9. We concluded strongly that the total time for cDNA synthesis should be completed not longer than 1 min by using a specific reverse primer set BQCV-VP1-R2.
Minimal detection time of Ultra-Rapid Real-Time PCR for BQCV detection from cDNA and specificity of BQCV detection primer
Because of high speed detection, we applied cDNA which was generated within 1 min using a reverse primer BQCV-VP1-R2 to URRT-PCR. The conditions were followed one pre-denaturation step at 95°C for 30 sec and 35 cycles of denaturation at 94, 95, 96°C for 1 sec, annealing at 62°C for 1 sec, extension at 72°C for 3 sec. The heating and cooling rate was 0.1°C/sec for all steps. The amplification of PCR products and a melting temperature analysis were clearly observed in Fig. 10. By URRT-PCR, BQCV can be detected completely from cDNA within 5 min 42 sec for 21 cycles and 8 min 8 sec for 30 cycles, excluding the loosing time for melting temperature analysis (Fig. 10, panel A, B).
To evaluate the specificity of BQCV-specific primer set, cDNAs from different kinds of virus-infected honeybees were tested by URRT-PCR following conditions: one predenaturation step at 95°C for 30 sec and 35 cycles of denaturation at 94°C for 1 sec, annealing at 62°C for 1 sec, extension at 72°C for 3 sec. The heating and cooling rate was 0.1°C/sec for all steps. The amplicon from URRTPCR of BQCV was compared to other viruses (Sacbrood bee virus and Israeli acute paralysis virus). Other virusinfected samples can’t be detected at all under the same condition, only BQCV was amplified from BQCV-cDNA which was confirmed before. It meant that the BQCV detection result from cDNA using this primer was accurate (Fig. 10, panel C, D).
For the diagnostic of viral diseases, a short reaction time may be a major requirement for the application of PCR analysis. Here, we developed a new protocol in which, RNA was rapidly extracted and high quality by one-step centrifugation at 13,000 rpm for 10 min at 4°C from cell lysate. This is the first modification from a general protocol of RNAiso Plus (Total RNA extraction reagent) (Takara, Japan) and give the good result in fact. Thus, we continued to minimizing the time in cDNA generation in each step. Instead of 60 min followed a general protocol using MMLV Reverse Transcriptase (Bioneer, Korea), the time was significantly dropped to 1 min and ready for qRT-PCR detection. Real-Time PCR has satisfied the requirements of a rapid detection method, such as accuracy, specificity, sensitivity and rapidity. Investigations on the sensitivity of qRT-PCR assay proved that low viral RNA copy number are detectable with this technique (Benjeddou et al., 2001), and studies on qRT-PCR verified that infected individual honeybees contain high viral titers (Chen et al., 2005a; Tentcheva et al., 2004). In our study, we used qRT-PCR to evaluate the sensitivity of detection primer sets: BQCVVP1-F2/BQCV-VP1-R2 and BQCV-VP3-F1/ BQCVVP3-R1, each of primer pairs could amplify amplicons even 103 and 102 DNA molecules. After 1 min cDNA generation, it was possible to apply directly this cDNA to a qRT-PCR detection and this reaction can be completed for 42 min corresponding to 19 cycles under an optimal condition. However, there were limitations existed in qRTPCR for the reduction of analysis time with a conventional real-time thermal cycler. To overcome this situation, we tried to introduce a microchip-based Real-Time thermal cycler, GenSpector® TMC-1000 (Samsung, Korea). This instrument facilitated to use by a computer analysis system, a micro-scale silicon-glass chip instead of plastic tubes and newly developed electronic temperature control modules. Therefore, the set reaction temperature of each cycle was rapidly reached because of high and stable thermal conductivity and small reaction volume (6μl/reaction). BQCV could be detected from cDNA amplified in URRTPCR within 5 min 42 sec for 21 cycles and 8 min 8 sec for 30 cycles. This time is also rapid compare to a previous research on BQCV detection using the same technique (Yoo et al., 2011) and studies on other viruses using URRT-PCR (Lim et al., 2013; Han et al., 2008). Moreover, URRT-PCR has shown that its high specificity for BQCV diagnosis since only cDNA from BQCV-infected honeybee sample could make a positive result otherwise cDNAs from IAPV or SBV infected honeybee samples did not gain any amplification. As a conclusion of this study, we thought if the whole viral detection process (from cDNA generation to PCR detection) could be completed within 10 min as our result (6 min 42 sec), it will be a valuable impact on disease control and the molecular diagnostics. The application of URRT-PCR is becoming useful for fast and reliable identification of BQCV or other pathogens and may be also helpful for studies of other diseases even in human.
Acknowledgments
This work was supported by Bio-Industry Technology Development Program (312027-3) and Advanced production technology Program (112042-03), ministry of Agriculture, Food and Rural Affairs, and Kyonggi University's Graduate Research Assistantship 2014.
LITERATURE CITED
-
Allen, M., and B. V. Ball, (1996), The incidence and world distribution of honey bee viruses, Bee World, 77, p141-162.
[https://doi.org/10.1080/0005772X.1996.11099306]
- Anderson, D.L., (1993), Pathogens and queen bees, Australian. Beekeeper, 94, p292-296.
-
Bailey, L., B. V. Ball, J. N. Perry, (1983), Association of viruses with two protozoal pathogens of the honey bee, Ann. Appl. Biol, 103, p13-20.
[https://doi.org/10.1111/j.1744-7348.1983.tb02735.x]
-
Bailey, L., R. D. Woods, (1977), Two more small RNA viruses from honey bees and further observations on sacbrood and acute bee-paralysis viruses, J. Gen. Virol, 37, p175-182.
[https://doi.org/10.1099/0022-1317-37-1-175]
-
Bakonyi, T., E. Grabensteiner, J. Kolodziejek, M. Rusvai, G. Topolska, W. Ritter, N. Nowotny, (2002), Phylogenetic analysis of acute bee paralysis virus strains, Appl. Environ. Microbiol, 68, p6446-6450.
[https://doi.org/10.1128/AEM.68.12.6446-6450.2002]
-
Benjeddou, M., N. Leat, M. Allsopp, S. Davison, (2001), Detection of acute bee paralysis virus and black queen cell virus from honeybees by reverse transcriptase PCR, Appl. Environ. Microbiol, 67, p2384-2387.
[https://doi.org/10.1128/AEM.67.5.2384-2387.2001]
-
Blanchard, P., M. Ribiere, O. Celle, P. Lallemand, F. Schurr, V. Olivier, A.L. Iscache, J.P. Faucon, (2007), Evaluation of a real-time two-step RT-PCR assay for quantitation of chronic bee paralysis virus (CBPV) genome in experimentally-infected bee tissues and in life stages of a symptomatic colony, J. Virol. Methods, 141(1), p7-13.
[https://doi.org/10.1016/j.jviromet.2006.11.021]
-
Chantawannakul, P., L. Ward, N. Boonham, M. Brown, (2006), A scientific note on the detection of honeybee viruses using real-time PCR (TaqMan) in Varroa mites collected from a Thai honeybee (Apis mellifera) apiary, 91, p69-73.
[https://doi.org/10.1016/j.jip.2005.11.001]
-
Chen, Y. P., J. A. Higgins, M. F. Feldlaufer, (2005a), Quantitative real-time reverse transcription-PCR ana-lysis of deformed wing virus infection in the honeybee (Apis mellifera L.), Appl. Environ. Microbiol, 71, p436-441.
[https://doi.org/10.1128/AEM.71.1.436-441.2005]
-
Cho, Y. K., J. T. Kim, Y. S. Lee, Y. A. Kim, (2006), Clinical evaluation of micro-scale chip-based PCR system for rapid detection of hepatitis B virus, Biosens. Bioelectron, 21, p2161-2169.
[https://doi.org/10.1016/j.bios.2005.10.005]
-
Han, S. H., D. B. Lee, D. W. Lee, E. H. Kim, B. S. Yoon, (2008), Ultra-rapid real-time PCR for the detection of Paenibacillus larvae, the causative agent of American Foulbrood (AFB), Invertebr Pathol, 99(1), p8-13.
[https://doi.org/10.1016/j.jip.2008.04.010]
- Huh, B., Y. J. Ha, J. T. Oh, E. H. Park, (2006), A rapid PCR-based assay for detecting hepatitis B viral DNA using GenSpector TMC-1000, Agric. Chem. Biotechnol, 49, p143-147.
- Kim, E. H., D. W. Lee, S. H. Han, Y. K. Lim, B. S. Yoon, (2007), Development of ultra-rapid multiplex real-time PCR for the detection of genes from avian influenza virus subtype H5N1, Korean J. Vet. Res, 47, p399-407.
-
Kricka, L. J., and P. Wilding, (2003), Microchip PCR, Anal. Bioanal. Chem, 377, p820-825.
[https://doi.org/10.1007/s00216-003-2144-2]
-
Leat, N., B. Ball, B. Govan, and S. Davison, (2000), Analysis of the complete genome sequence of black queen cell virus, a picorna-like virus of honey bees, Journal of General Virology, 81, p2111-2119.
[https://doi.org/10.1099/0022-1317-81-8-2111]
- Lee, D. W., E. H. Kim, M. S. Yoo, S. H. Han, B. S. Yoon, (2007), Ultra-rapid real-time PCR for the detection of human immunodeficiency virus (HIV), Kor. J. Microbiol, 43, p91-99.
- Lim, H. Y., and B. S. Yoon, (2013), Rapid and sensitive detection of Deformed Wing Virus (DWV) in honeybee using Ultra-rapid Real-time PCR, Kor. J. Apicul, 28(2), p121-129.
- Mello, A. J. D., (2001), DNA amplification: does small really mean efficient, Lab Chip, 1, p24N-29N.
- Shimanuki, H., N. W. Calderone, D. A. Knox, (1994), Parasitic mite syndrome: the symptoms, Am. Bee J, 134, p827-828.
-
Tentcheva, D., L. Gauthier, S. Jouve, L. Canabady-Rochelle, B. Dainat, F. Cousserans, M. E. Colin, B. V. Ball, M. Bergoin, (2004), Polymerase chain reaction detection of deformed wing virus (DWV) in Apis mellifera and Varroa destructor, Apidologie, 35, p431-439.
[https://doi.org/10.1051/apido:2004021]
-
Tentcheva, D., L. Gauthier, N. Zappulla, B. Dainat, F. Cousserans, M. E. Colin, M. Bergoin, (2004), Prevalence and seasonal variations of six bee viruses in Apis mellifera L. and Varroa destructormite populations in France, Appl. Environ.Microbiol, 70, p7185-7191.
[https://doi.org/10.1128/AEM.70.12.7185-7191.2004]
- Topley, E., S. Davison, N. Leat, M. Benjeddou, (2005), Detection of three honeybee viruses simultaneously by a single multiplex reverse transcriptase PCR, Afr. J. Biotechnol, 4, p763-767.
- Yoo, M. S., S. H. Han, B. S. Yoon, (2011), Development of Ultra-Rapid Real-time PCR method for detection of Black Queen Cell Virus, Kor. J. Apicul, 26(3), p203-208.