
Further Finding of Antimicribial and Antifungal Activities against Human Pathegens by using the Isolates to Inhibit the Growth of Paenibacillus lavae, an Agent of AFB
Abstract
More than 100 soil samples were collected from many forests in Korea during 2013. We found 43 strains from more than 2,000 isolates, which showed antimicrobial activity against Paenibacillus larvae as an agent of American Foulbrood (AFB) as well as common microbial human pathogens such as Bacillus subtilis, Staphylococcus aureus, Escherichia coli, Candida albicans and Aspergillus niger. On the basis of 16S rRNA gene sequence analysis, they belong to 12 genera (mostly actinobacteria): Streptomyces (28), Kitasospora (4), Bacillus (2), Streptacidiphilus, Amycolatopsis, Paenibacillus, Promicromonospora, Rhodococcus, Pseudomonas, Burkholderia, Undibacterium and Actinomadura. Among them, Streptomyces species are dominant (28/43 strains, 65.12%). Burkholderia and Undibacterium spp. were reported first as antimicrobial agents, especially against Gram-positive bacteria. In the future, they may be useful to develop some drugs against human pathogens by using their metabolites.
Keywords:
Paenibacillus larvae, American Foulbrood disease (AFB), Actinobacteria, Human pathogens, AntibioticsINTRODUCTION
Microorganisms participate in almost all metabolic processes of life and make up 60% of the earth'sbiomass (Singh et al., 2009). Among them, many ones can produce lots of useful metabolic products such as antibiotics. Penicillin, discovered by Alexander Fleming in 1928, paved the way for a golden era of natural antibiotics in the 1940~1950s. However, any substrates to replace these antibiotics have not been found until now. The number of newly discovered antibiotics had decreased in the later years, but recently, advances in technology and screening methods enable the detection of previously unidentified compounds (Kurtboke, 2012). For example, some novel antibiotics were identified from different strains of the same species that shares 99.6~100% similarity of 16S rRNA sequence (Tamehiro et al., 2002; Nachtigall et al., 2011) and from new species or new genus (Clardy et al., 2006; Lewis, 2013; Ling et al., 2015).
Paenibacillus larvae (P. larvae) is an agent of American Foulbrood disease (AFB) and a spore-forming Grampositive bacterium. Adult bees can be resistant to this bacterial disease, but bee larvae are very susceptible to infection (Wilson, 1971; Hitchcock et al., 1979). Because spores of P. larvae endure against heating, some antibiotics and other chemical agents (Genersch, 2010), the spores can survive for long time in environments or regional beekeeping hives (up to 35 years) (Heyndrickx et al., 1996; Dobbelaere et al., 2001).
This study extended to screen our isolates with some microbial pathogens causing popular diseases such as Bacillus subtilis, Escherichia coli, Staphylococcus aureus, Aspergillus niger, and Candida albicans. B. subtilis is well known as an agent of endocarditis, bacteraemia, pneumonia, septicaemia (Pennington et al., 1976), and carcinoma of the breast (Ihde and Armstrong, 1973). Furthermore, B. subtilis spores seems to survive against heat treatment and amylase activity (Pepe et al., 2003) and to present with high amounts in some foods (106 c.f.u/g) (Gilbert et al., 1981). Like B. subtilis, A. niger can produce the spores that can exist for a long period, and is known as a cause of pneumonia's desease (Person et al., 2010). C. albicans is the main agent of fungal pathogens in humans, especially for immunocompromised patients (Tsai et al., 2013). Recently, there is an evidence that C. albicans may be a dangerous agent for cancer patients since they promote division and differentiation of cancer cells (Ramirez-Garcia et al., 2014). Unlike pathogenic microorganisms mentioned above, Escherichia coli species are "useful" intestinal bacteria because they participate in digesting foods. However, there are three types of desease caused by them, which are enteric/diarrhoeal diseases, urinary tract infections and sepsis/meningitis (Kaper et al., 2004). S. aureus is a common type of bacteria that lives on the skin and mucous membranes of humans, causing various human's skin diseases. The cells have the capsid that protects them from destruction of phagocytes and supports in attachment to various host tissues (Dunman and Projan, 2002).
Since antibiotics were used in the world war II for effective treatment of infectious diseases, many researchers have reported from the beginning of the 1950’s that some pathogens were resistant to antibiotics known (Madigan et al., 2009). For example, they were methicillin-resistant Staphylococcus aureus, vancomycin-resistant Enterococcus, and vancomycin-resistant Staphylococcus aureus (Klevens et al., 2006; Moran et al., 2006). Further more, Candida albicans was resistant to all known antimicrobial drugs (Madigan et al., 2009). Therefore, researchers need to discover novel antibiotics against the resistant microbial pathogens (Donadio et al., 2010).
Actinobacteria is Gram-positive bacteria with high DNA G+C contents (60~78 mol%), can produce a wide variety and the most important secondary metabolites that can be applied in industrial medicine and therapeutic diseases (Baltz, 2005; Rokem et al., 2007). Those are antibacterial, anticancer, antitumor, antiinfective, antiinflammatory and antifungal agents (Shiomi and Omura, 2004; Caffrey et al., 2008; Baltz, 2008; Graziani, 2009; Olano et al., 2009). Actinobacteria can be found in terrestrial, freshwater and marine ecosystems, but more abundant in soil (Gremion et al., 2003; Janssen, 2006). The genus Streptomyces was first described by Waksman & Henrici (1943), widely distributed in nature. In soil, Streptomyces species were isolated with abundant nummbers, containing 1~20% (104 ~ 107 c.f.u/g) of the total number of colonies that can be counted on petri disk (Korn-Wendisch and Kutzner, 1992). Streptomyces account for over 74% of the antibiotics produced by the order actinomycetales, and approximately 34% of all known antibiotics (Berdy, 2005). Streptomyces species were described as the richest in the prokaryotic including over 668 species with valid names (http://www.bacterio.net/streptomycesa.html). Hence the number of Streptomyces species in this study was dominant.
However, according to Pham and Kim (2012), the majority of microorganisms (more than 99%) can not grow in laboratory conditions and so their phenotypic characteristics have not been detected. Therefore, we applied three methods including a new method using transwell plates for isolation of new actinobacteria and other bacteria including not-yet-cultured strains in this study, and then screened all the isolates to find the strains to have antimicribioal activity against P. lavae and human pathogens.
MATERIALS AND METHODS
Isolation of actinobacteria
We used three methods for isolation of soil actinobacteria and other bacteria as described previously (Nguyen et al., 2013). Method 1 was developed by Pham and Kim (2014) to isolate a variety of soil bacteria which may be unculturable or hardly cultivated. Some samples were treated at 40°C for 16 h to isolate common actinobacteria (Method 2: Williams and Wellington, 1982) and to isolate rare actinobacteria 30 min at 120°C (Method 3: Nonomura and Ohara, 1971).
Test microorganisms
Microbial pathogens were obtained from the Korean Agricultural Culture Collection (KACC) and the Korea National Environmental Microorganisms Bank (KEMB). These bacteria included Paenibacillus larvae KACC 14031, Bacillus subtilis KEMB 51201-001, Staphylococcus aureus KEMB 4659, Escherichia coli KEMB 212-234, Candida albicans KACC 30003 and Aspergillusniger KACC 40280.
Assay of antibacterial and antifungal activities
For preparing microbial pathogens, the bacteria in glycerol stock were refreshed in 20mL of LB medium (except P. larvae with R2A) at optimal temperature overnight and then streaked on agar plates. Single colonies were picked and adjusted to 104 c.f.u/mL with R2A broth. Spores of A. niger were prepared at 104 spores/mL, and spores were taken from Sabaroud dextrose agar. C. albicans was grown on YM medium (ATCC medium 200) at 104 c.f.u/mL. The absorbance of the suspensions was measured at 550 nm for fungal spores and 600 nm for bacterial cells. Then, 100μL of bacterial suspension or spore suspension was spread on R2A agar plates (petri dish size, 90×15mm). Pure colonies of isolates were picked off and spotted on the plates on which the human pathogens were spread in advance. These agar plates were incubated at 28°C and then the diameter of inhibition zone was measured after incubation for 3~4 days.
Identification and phylogenetic anlaysis by 16S rRNA gene sequence comparison
The almost-complete 16S rRNA gene sequences of our strain were identified by using the EzTaxon-e server (http://www.ezbiocloud.net/eztaxon). The 16S rRNA gene sequences of related taxa were obtained from GenBank and edited using the BioEdit program (Hall, 1999). Multiple alignments were performed with the CLUSTAL_ X program (Thompson et al., 1997). Evolutionary distances were calculated using the Kimura two parameter model (Kimura, 1983). Phylogenetic trees were constructed using the neighbour-joining method with the MEGA5.03 program (Tamura et al., 2011); bootstrap values were based on 1,000 replicates (Felsenstein, 1985).
RESULTS AND DISCUSSION
In this study, we found 43 strains that inhibited the growth of Paenibacillus larvae. Those strains were obtained through screening more than 2,000 isolates which were isolated from 100 different soil samples collected from all around South Korea. The number of highly active strains (the diameter of inhibition zone ≥ 20mm) were 9 (20.93%) (Table 1). Especially, Bacillus sp. T515 (96.72% similarity) proven to be a new species (Nguyen and Kim, 2015) can be a strong candidate to discover new antibiotics. Although others have the values between 99.18 to 100% in similarity levels of 16S rRNA gene sequences with the closest species (except strain Bacillus sp. T515), they were still potential candidates for discovering new antibiotics as mentioned before. Besides, there were two other strains that may be new species [Streptomyces sp. T523 (98.75% similarity) and Undibacterium sp. T913 (98.05% similarity)] which can be candidates to discover new antibiotics, in spite of less active strains (12 and 8 mm in inhibition zone, respectively).

List of actinobacterial and other bacterial strains that showed antibacterial and antifungal activities against human pathogens by using anti-P. larve isolates. 1: Paenibacillus larvae KACC 14031; 2: Bacillus subtilis KEMB 51201-001; 3: Staphylococcust aureus KEMB 4659; 4: Escherichia coli KEMB 212-234; 5: Candida albicans KACC 30003; 6: Aspergillus niger KACC 40280
Among the effective 43 strains against P. larvae, 23 strains (53.49%) showed inhibitory activity against Staphylococcus iaureus, 22 strains (51.16%) against Bacillus subtilis, 15 strains (34.88%), against Aspergillus niger, 12 strains (27.91%) against Escherichia coli, and 12 strains against Candida albicans (Fig. 1). The number of strains showing high activity (≥ 20mm in inhibition zone) against at least one of human pathogens was 5 among 43 (11.63%). They are Bacillus sp. T515 against S. aureus, Streptomyces sp. T525 against B. subtilis and S. aureus, Streptomyces sp. T804 against E. coli, Streptomyces sp. T806 against E. coli, and Kitasatospora sp. T1305 against C. albicans (Table 1). It is considered to be potential strains for producing useful compounds as effective antibiotics than any other strains. Althogh many other strains showed low activity, they may be increased in acitivity by better expression of their functional genes through optimal culture conditions. For example, during the screening process, Bacillus sp. T515 was effective on only P. larvae, B. subtilis and S. aureus (all Gram-positive), but the spectrum of control was extended further by choosing an optimal medium. Its crude extractant could kill both Gramnegative bacteria (Escherichia coli and Pseudomonas aeruginosa) and Gram-positive bacteria (Bacillus subtilis, Staphylococcus aureus, Staphylococcus epidermidis, Paenibacillus larvae) (Nguyen and Kim, 2015).
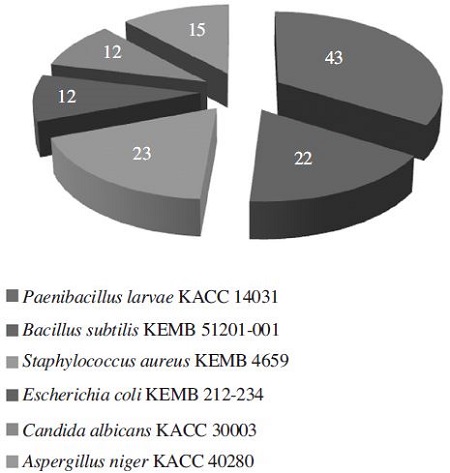
Distribution (number among total 43 strains) of the strains showing activities against microbial pathogens, isolated from soil samples collected in South Korea.
However, it seems to be very hard to find bacterial strains to control a broad spectrum of infectious human pathogens including prokaryotic Gram-negative and Grampositive bacteria and eukaryotic fungi. Only three strains among 43 can control three different groups (G+-bacteria, G--bacteria and fungi) such as Streptomyces spp. T82, T806 and T822. In particular, strain T82 showed inhibitory effect on all the tested pathogens (Table 1).
On the basis of 16S rRNA sequence analysis, the 43 strains belong to 12 genera: 7 genera in actinobacteria (Streptomyces, Kitasospora, Streptacidiphilus, Amycolatopsis, Promicromonospora, Rhodococcus and Actinomadura) and 5 genera in other bacteria (Bacillus, Pseudomonas, Burkholderia, Undibacterium and Paenibacillus) (Fig. 2). Among 43 strains, 28 (65.12%) were identified as the genus Streptomyces, a most well-known antibioticproducing genus (Berdy, 2005). Although they were proven to have a little possibility of new species (98.75~100% similarity to type species, most of which were discovered in the 1950s), they are still potential candiates to produce new secondary active metabolites because they were not discovered during that period mentioned above. Among the rest 11 genera, Kitasospora and Bacillus had 4 and 2 strains, respectively, and other genera had 1 each. Nonactinobacterial genera can be divided into two groups, Gram-positive (Bacillus and Paenibacillus) and Gramnegative (Pseudomonas, Burkholderia and Undibacterium). Phylogenetic analysis of our strains were constructed to establish their relationship. Based on the analysis of 16S rRNA gene sequences, they were divided into three groups in the level of phylum such as actinobacteria (major), fermicutes and proteobacteria (Fig. 3). Actinobacteria and fermicutes can be bound in Gram-positive bacteria, separating from Gram-negative proteobacter.
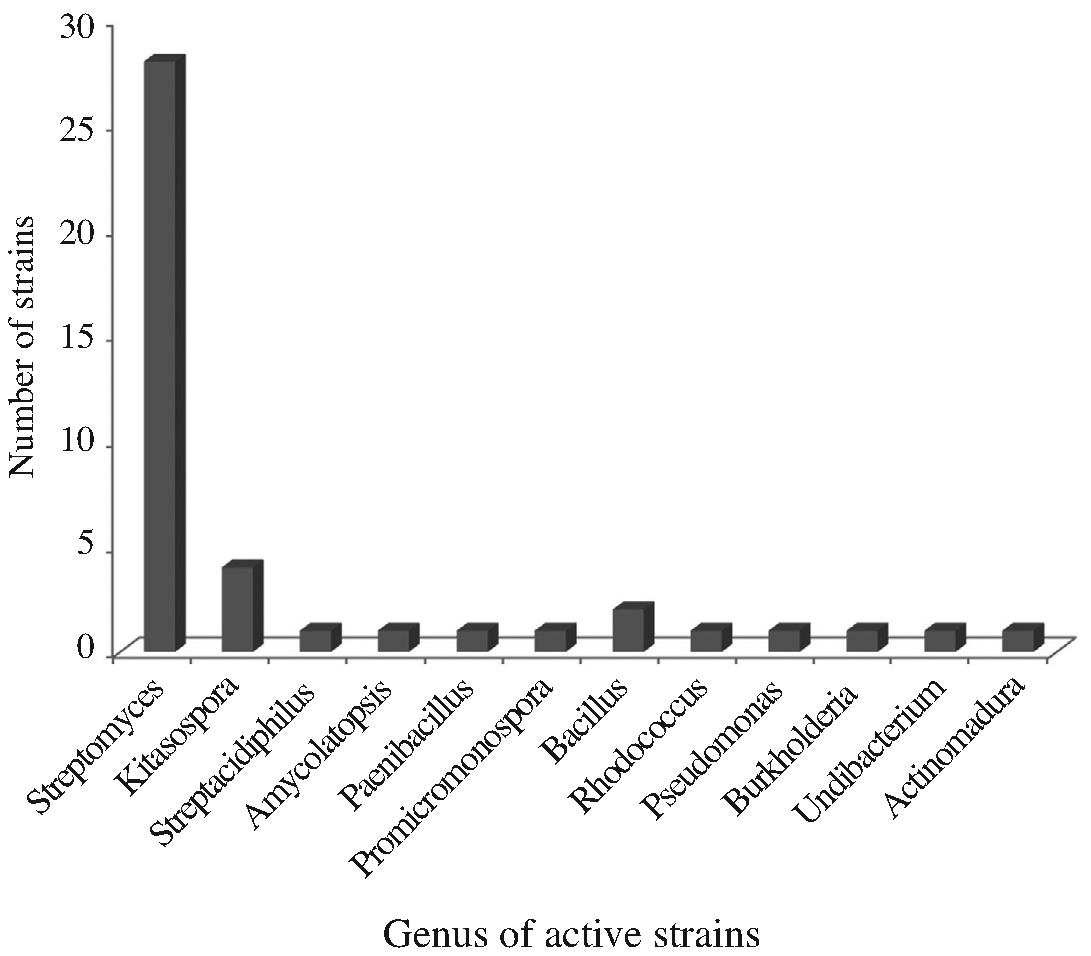
Relative distribution of the active strains in the level of bacterial genus, showing antimicrobial and antifugal activities against microbial pathogens.
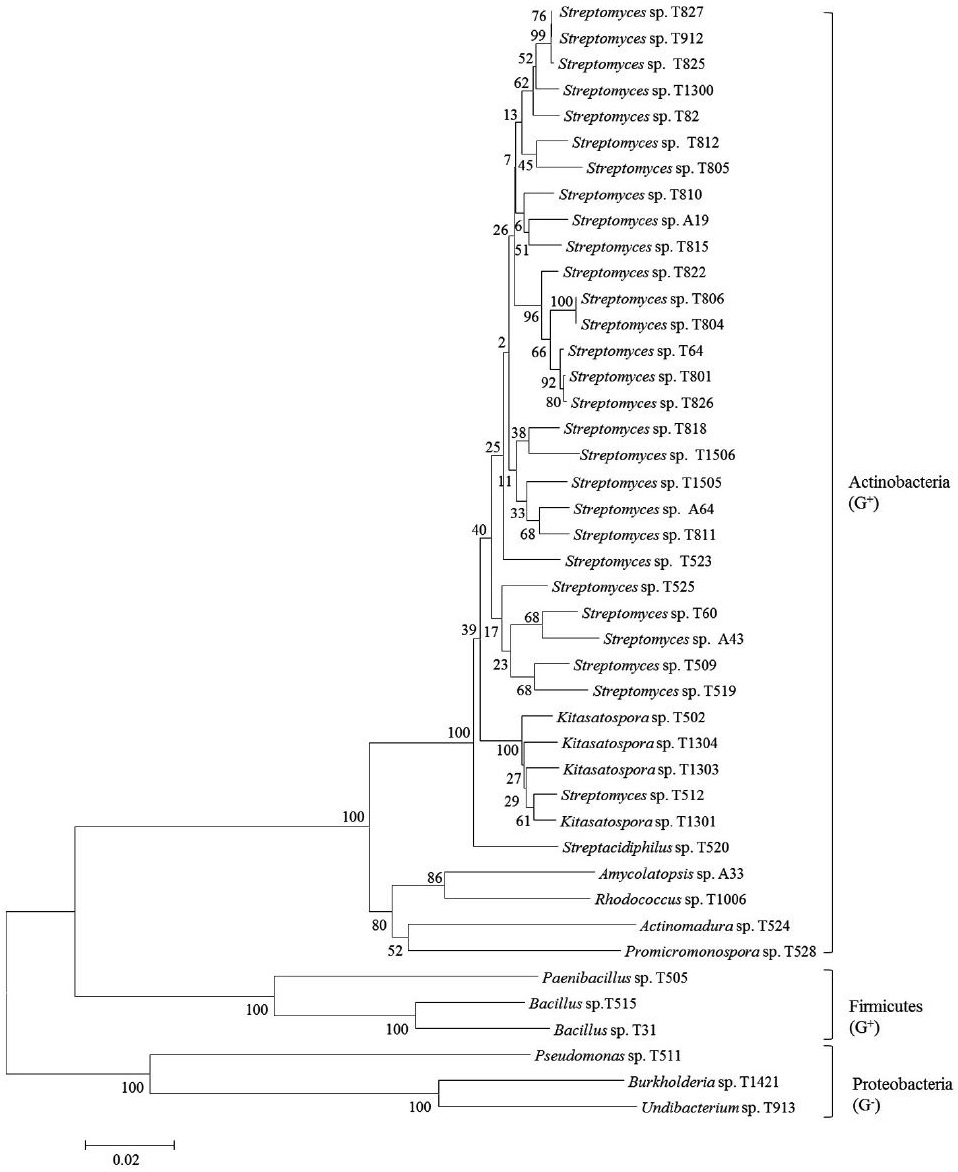
A phylogenetic tree of selected antimicrobial strains isolated from various soil samples based on 16S rRNA gene sequence analysis.
In conclusion, natural balances are always in a state of activity, and so effect of climate change and others factors increase the evolution of microorganisms. Since antibiotics had discovered and used up to today, lots of microbial pathogens have developed their resistancy to various antibiotics. Hence we have conducted to find new candidates that may be the sources to control antibiotic-resistant P. larvae and human pathogens in this study. As a result, we have recruited a number of species including Grampositive and Gram-negative bacteria, which are the candidates for a research of novel antibiotics/secondary metabolites. A continuous study will be performed for isolation and purification of new compounds, and examination of their activity against antibiotic-resistant pathogens.
Acknowledgments
This study was supported by Bio-industry Technology Development Program (312027-3), Ministry of Agriculture, Food and Rural Affairs, by the Basic Science Research Program through the National Research Foundation of Korea (NRF), funded by the Ministry of Education, Science, and Technology (2011-0010144), and also by Kyonggi University Research Assistant Fellowship 2015.
LITERATURE CITED
- Baltz, R. H., (2005), Antibiotic discovery from actinomycetes: will a renaissance follow thedecline and fall?, Sim News, 55, p186-96.
-
Baltz, R. H., (2008), Renaissance in antibacterial discovery from actinomycetes, Curr. Opin. Pharmacol, 8, p557-563.
[https://doi.org/10.1016/j.coph.2008.04.008]
-
Berdy, J., (2005), Bioactive microbial metabolites, J. Antibiot, 58, p1-26.
[https://doi.org/10.1038/ja.2005.1]
-
Caffrey, P., J. F. Aparicio, F. Malpartida, and S. B. Zotchev, (2008), Biosynthetic engineering of polyene macrolides towards generation of improved antifungal and antiparasitic agents, Curr. Top. Med. Chem, 8, p639-653.
[https://doi.org/10.2174/156802608784221479]
-
Clardy, J., M. A. Fischbach, and C. T. Walsh, (2006), New antibiotics from bacterial natural products, Nature biotechnology, 24, p1541-1550.
[https://doi.org/10.1038/nbt1266]
-
Dobbelaere, W., D. C. De Graaf, W. Reybroeck, E. Desmedt, J. E. Peeters, and F. J. Jacobs, (2001), Disinfection of wooden structures contaminated with Paenibacillus larvae subs P. larvae spores, J. Appl. Microbiol, 91, p212-216.
[https://doi.org/10.1046/j.1365-2672.2001.01376.x]
-
Donadio, S., S. Maffioli, P. Monciardini, M. Sosio, and D. Jabes, (2010), Antibiotic discovery in the twenty-first century: current trends and future perspectives, J. Antibiot, 63, p423-430.
[https://doi.org/10.1038/ja.2010.62]
- Dunman, P. M., and S. J. Projan, (2002), The Regulation of virulence in the Staphylococci, p4, in Staphylococcus aureus infection and disease, eds. by A. L. Honeyman, H. Friedman, and M. Bendinelli, New York, Boston, Dordrecht, London, Moscow.
-
Felsenstein, J., (1985), Confidence limit on phylogenies: an approach using the bootstrap, Evolution, 39, p783-791.
[https://doi.org/10.2307/2408678]
-
Genersch, E., (2010), American foulbrood in honeybees and its causative agent, Paenibacillus, J. Invertebr. Pathol, 103, p510-519.
[https://doi.org/10.1016/j.jip.2009.06.015]
- Gilbert, R. J., P. C. B. Turnbull, J. M. Parry, J. M. Kramer, (1981), Bacillus cereus and other Bacillus species : their part in food poisoning and other clinical infections, p297-314, in The aerobic endospore-forming bacteria ; classification and identification, eds. by R. C. W. Berkeley, and M. Goodfellow, Academic Press, London.
-
Graziani, E. I., (2009), Recent advances in the chemistry, biosynthesis and pharmacology of Rapamycin analogs, Nat. Prod. Rep, 26, p602-609.
[https://doi.org/10.1039/b804602f]
-
Gremion, F., A. Chatzinotas, and H. Harms, (2003), Comparative 16S rDNA and 16S rRNA sequence analysis indicates that Actinobacteria might be a dominant part of the metabolically active bacteria in heavy metalcontaminated bulk and rhizosphere soil, Environ. Microbiol, 5, p896-907.
[https://doi.org/10.1046/j.1462-2920.2003.00484.x]
- Hall, T. A., (1999), BioEdit: A user-friendly biological sequence alignment editor and analysis program for Windows 95/98/NT, Nucleic. Acids. Symp. Ser, 41, p95-98.
-
Heyndrickx, M., K. Vandemeulebroecke, and B. Hoste, et al , (1996), “Reclassification of Paenibacillus (formerly Bacillus) pulvifaciens (Nakamura 1984) Ash et al. 1994, a later subjective synonym of Paenibacillus (formerly Bacillus) larvae (White 1906) Ash et al. 1994, as a subspecies of P. larvae, with emended descriptions of P. larvae as P. larvae subs P. larvae and P. larvae subsp. pulvifaciens, Int. J. Syst. Bacteriol, 46, p270-279.
[https://doi.org/10.1099/00207713-46-1-270]
- Hitchcock, J. D., A. Stoner, W. T. Wilson, and D. M. Menapace, (1979), Pathogenicity of Bacillus pulvifaciens to honeybee larvae of various ages (Hymenoptera: Apidae), J. Kansas Entomol. Soc, 52, p238-246.
-
Ihde, D. C., and D. Armstrong, (1973), Clinical spectrum of infection due to Bacillus species, American Journal of Medicine, 55, p839-845.
[https://doi.org/10.1016/0002-9343(73)90266-0]
-
Janssen, P. H., (2006), Identifying the dominant soil bacterial taxa in libraries of 16S rRNA and 16S rRNA genes, Appl. Environ. Microbiol, 72, p1719-1728.
[https://doi.org/10.1128/AEM.72.3.1719-1728.2006]
- Kaper, J. B., J. P. Nataro, and H. L. T. Mobley, (2004), Pathogenic Escherichia coli, Nature reviews, 2, p123-140.
-
Kimura, M., (1983), The Neutral Theory of Molecular Evolution, Cambridge University Press, Cambridge, UK.
[https://doi.org/10.1017/CBO9780511623486]
-
Klevens, R. M. J. R., F. C. Edwards, L. C. Tenover, T. McDonald, R. Horan, R. Gaynes, and , National Nosocomial Infections Surveillance System, (2006), Changes in the epidemiology of methicillin-resistant Staphylococcus aureus in intensive care units in US hospitals, 1992-2003, Clin. Infect. Dis, 42, p389-391.
[https://doi.org/10.1086/499367]
- Korn-Wendisch, F., and H. J. Kutzner, (1992), The family Streptomycetaceae, p921-995, in The Prokaryotes: a Handbook on the Biology of Bacteria: Ecophysiology, Isolation, Identification, Applications, eds. by Balows, Truper, Dworkin, Harder, and Schleifer, 2nd ed, Springer, New York.
-
Kurtboke, D. I., (2012), Biodiscovery from rare actinomycetes: an eco-taxomical perspective, Appl. Microbiol. Biotechnol, 93, p1843-1852.
[https://doi.org/10.1007/s00253-012-3898-2]
- Lewis, K., (2013), Platforms for antibiotic discovery, Nature reviews, 12, p371-387.
-
Ling, L. L., T. Schneider, A. J. Peoples, A. L. Spoering, I. Engels, B. P. Conlon, A. Mueller, and other. , (2015), A new antibiotic kills pathogens without detectable resistance, Nature, 517, p455-459.
[https://doi.org/10.1038/nature14098]
- Madigan, M. T., J. M. Martinko, P. V. Dunlap, and D. P. Clark, (2009), Metabolic diverity and microbial ecology, p805-806, in Brock Biology of Microorganisms (12th ed). Pearson international edition.
-
Moran, G. J., A. Krishnadasan, R. J. Gorwitz, G. E. Fosheim, L. K. McDougal, and others , (2006), Methicillin-resistant Staphylococcus aureus infections among patients in the emergency department, N. Engl. J. Med, 355, p666-674.
[https://doi.org/10.1056/NEJMoa055356]
-
Nachtigall, J., K. Schneider, C. Bruntner, A. T. Bull, M. Goodfellow, H. Zinecker, J. F. Imhoff, G. Nicholson, E. Irran, R. D. Sussmuth, and H. P. Fiedler, (2011), Benzoxacystol, a benzoxazine-type enzyme inhibitor from the deep-sea strain Streptomyces sp. NTK 935, J. Antibiot, 64, p453-457.
[https://doi.org/10.1038/ja.2011.26]
-
Nguyen, T. M., and J. Kim, (2015), Bacillus polymachus sp. nov., with a broad range of antibacterial activity, isolated from forest topsoil samples by using a modified culture method, Int. J. Syst. Evol. Microbiol, 65, p704-709.
[https://doi.org/10.1099/ijs.0.070326-0]
- Nguyen, T. M., H. Lee, and J. Kim, (2013), Selective isolation of actinobacteria showing antibacterial activity against Paenibacillus from soil samples collected in South Korea, J. Apiculture, 28, p265-272.
- Nonomura, H., and Y. Ohara, (1971), Distribution of actinomycetes in soil. A new genus and species of monosporic actinomycetes in soil, J. Ferment. Technol, 49, p895-903.
-
Olano, C., C. Mendez, and J.A. Salas, (2009), Antitumor compounds from marine actinomycetes, Mar. Drugs, 7, p210-248.
[https://doi.org/10.3390/md7020210]
-
Pennington, J. E., N. D. Gibbons, J. E. Strobeck, G. L. Simpson, and R. L. Myerowitz, (1976), Bacillus species infection in patients with hematologic neoplasia, Journal of the American Medical Association, 235, p1473-1474.
[https://doi.org/10.1001/jama.1976.03260400039027]
-
Pepe, O., G. Blaiotta, G. Moschetti, T. Greco, and F. Villan, (2003), Rope-Producing strains of Bacillus spp. from wheat bread and strategy for their control by lactic acid bacteria, Appl. Environ. Microbiol, 69, p2321-2329.
[https://doi.org/10.1128/AEM.69.4.2321-2329.2003]
-
Person, A. K., S. M. Chudgar, B. L. Norton, B. C. Tong, and J. E. Stout, (2010), Aspergillus niger: an unusual cause of invasive pulmonary aspergillosis, Journal of Medical Microbiology, 59, p834-838.
[https://doi.org/10.1099/jmm.0.018309-0]
-
Pham, V. H. T., and J. Kim, (2014), Bacillus thaonhiensis sp. nov., a new species, was isolated from the forest soil of Kyonggi University by using a modified culture method, Curr. Microbiol, 68, p88-95.
[https://doi.org/10.1007/s00284-013-0443-1]
-
Pham, V. H. T., and J. Kim, (2012), Cultivation of unculturable soil bacteria, Trends. Biotechnol, 30, p475-484.
[https://doi.org/10.1016/j.tibtech.2012.05.007]
-
Ramirez-Garcia, A., A. Rementeria, J. M. Aguirre-Urizar, M. D. Moragues, A. Antoran, A. Pellon, A. Abad-Diaz-de-Cerio, and F. L. Hernando, (2014), Candida albicans and cancer: Can this yeast induce cancer development or progression?, Crit. Rev. Microbiol, p1-13.
[https://doi.org/10.3109/1040841X.2014.913004]
-
Rokem, J. S., A. E. Lantz, and J. Nielsen, (2007), Systems biology of antibiotic production by microorganisms, Nat. Prod. Rep, 24, p1262-1287.
[https://doi.org/10.1039/b617765b]
-
Shiomi, K., and S. Omura, (2004), Antiparasitic agents produced by microorganisms, Proc. Jpn. Acad. Ser. B. Phys. Biol. Sci, 80, p245-258.
[https://doi.org/10.2183/pjab.80.245]
-
Singh, B. K., C. D. Campbell, S. J. Sorenson, and J. Zhou, (2009), Soil genomics, Nat. Rev. Microbiol, 7, p756.
[https://doi.org/10.1038/nrmicro2119-c1]
-
Tamehiro, N., Y. O. Hosoya, S. Okamoto, M. Ubukata, M. Hamada, H. Naganawa, and K. Ochi, (2002), Bacilysocin, a Novel Phospholipid Antibiotic Produced by Bacillus subtilis 168, Antimicrob. Agents. Chemother, 46, p315-320.
[https://doi.org/10.1128/AAC.46.2.315-320.2002]
-
Tamura, K., D. Peterson, N. Peterson, G. Stecher, M. Nei, and S. Kumar, (2011), MEGA 5: Molecular evolutionary genetics analysis using maximum likelihood, evolutionary distance, and maximum parsimony methods, Mol. Biol, 28, p2731-2739.
[https://doi.org/10.1093/molbev/msr121]
-
Thompson, J. D., T. J. Gibson, F. Plewniak, F. Jeanmougin, and D. G. Higgins, (1997), The CLUSTAL_X windows interface: flexible strategies for multiple sequence alignment aided by quality analysis tools, Nucleic. Acids. Res, 25, p4876-4882.
[https://doi.org/10.1093/nar/25.24.4876]
-
Tsai, P. W., Y. T. Chen, P. C. Hsu, and C. Y. Lan, (2013), Study of Candida albicans and its interactions with the host: A mini review, Bio Medicine, 3, p51-64.
[https://doi.org/10.1016/j.biomed.2012.12.004]
- Waksman, S. A., and A. T. Herici, (1943), The nomenclature and classification of the actinomycetes, J. Bacteriol, 46, p337-341.
- Williams, S. T., and E. M. H. Wellington, (1982), Actinomycetes, p969-987, in Methods of soil analysis, part 2: Chemical and Microbiological Properties, eds. by A.L. Page, R. H. Miller, and O. R. Keency, 2nd ed, American Society of Agronomy/Soil Science Society of America, Madison.
-
Wilson, W. T., (1971), Resistance to American foulbrood in honey bees XI. Fate of Bacillus larvae spores ingested by adults, J. Invertebr. Pathol, 17, p247-255.
[https://doi.org/10.1016/0022-2011(71)90099-1]