
Diversity and Dynamics: Virome Analysis of Two Honey Bee Species across Pakistan and Bangladesh
Abstract
Honey bee viruses remain largely unexplored in Pakistan and Bangladesh, where commercial apiculture plays an essential role in agricultural and economic sustainability. This study presents a comprehensive virome analysis of two key honey bee species, Apis mellifera and A. cerana, from these regions, utilizing high-throughput sequencing (HTS) technology. Our findings reveal the presence of nine honey bee viruses and seven plant viruses, including four novel viruses. Notably, Apis mellifera-associated partiti-like virus 2, first identified in South Korea, was consistently detected in all samples from Pakistan. Additionally, we identified a novel virus exhibiting minimal similarity to known members of the Tobamovirus and Alphaendornavirus genera. Moreover, we confirmed the complete genome sequence of Apis mellifera filamentous virus in A. mellifera samples from Bangladesh. This pioneering virome analysis highlights previously undetected viruses in honey bee populations of Pakistan and Bangladesh, providing critical insights for future research on honey bee health and viral ecology in these regions.
Keywords:
Apis mellifera, Apis cerana, High-throughput sequencing, Virome analysis, Honey bee virus, Pakistan, BangladeshINTRODUCTION
The Apis mellifera species is globally cultivated as a pollinator for numerous crops and is also economically significant to produce industrial goods like honey, royal jelly, wax, and propolis (Popovska Stojanov et al., 2021; Zacepins et al., 2021). A. cerana remains primarily managed in Asian countries (Theisen-Jones and Bienefeld, 2016). Both species are under constant threat from diseases, environmental changes, and pesticides, significantly impacting bee health and stability of bee colonies (Atkins, 1992; Allen and Ball, 1996; Le Conte and Navajas, 2008). A prominent issue for A. mellifera is Colony Collapse Disorder (CCD), which has led to considerable population declines worldwide. A. cerana, in contrast, has faced notable mortality from sacbrood virus (SBV) outbreaks in countries such as Korea, Vietnam, Thailand, India, and China (Bailey et al., 1983, Liu et al., 2010, Choe et al., 2012; Nguyen and Le, 2013).
Virological research on honey bees initially lagged behind other fields, with sacbrood virus identified as the first known honey bee virus (White, 1913). Interest in honey bee virology surged following the CCD phenomenon in 2007, with the number of identified viruses rising from just 17 prior to CCD to over 128 by 2023 (Chen and Siede, 2007; Oldroyd, 2007; Beaurepaire et al., 2020; Kwon et al., 2023a). Despite these advances, significant gaps remain, particularly in regions like Pakistan and Bangladesh, where honey bees are extensively managed but relatively under-researched.
Beekeeping in Pakistan mainly involves the commercial beekeeping of two species, A. mellifera and A. cerana, with recent estimates indicating approximately 600,000 colonies managed by 10,000 beekeepers, producing about 12,000 tons of honey annually (Khan and Ghramh, 2023). Alongside virus threats, honey bees in Pakistan face various pests and diseases, including Varroa destructor, Tropilaelaps clareae, American foulbrood (AFB), and chalkbrood (CB) (Waghchoure-Camphor and Martin, 2009; Rehman et al., 2018; Khan and Ghramh, 2023). In Bangladesh, beekeeping encompasses five species, of which four (A. cerana, A. dorsata, A. florea, Trigona sp.) are native, including A. cerana (TBS, 2020). In 2023 survey, commercial apiculture is projected to involve around 70,000 colonies, maintained by 12,000-15,000 beekeepers with an annual honey yield of 5,000 tons (Haque, 2023). However, studies on honey bee pests and pathogens in Bangladesh are still limited.
Advancements in sequencing technologies, from Sanger sequencing to second-generation high-throughput sequencing (HTS), have greatly expanded virological research by reducing analysis time and costs (Collins et al., 2003; Mardis, 2011; Pareek et al., 2011). Additionally, several thirdand fourth-generation analysis platforms are now in use (Feng et al., 2015; Rhoads and Au, 2015; Deamer et al., 2016). These advancements have significantly reduced the extensive time required for analysis by Sanger sequencing and the cost of analysis by more than 100-fold (Schloss, 2008). HTS has catalyzed progress in bioinformatics, especially virome analysis, which comprehensively examines viral communities in a sample, including known, novel, and asymptomatic viruses (Ercolini, 2013; Kapoor and Lipkin, 2014; Conesa et al., 2016; Sekse et al., 2017; Kwon et al., 2023b). In honey bees, virome analysis has illuminated the viral diversity affecting bees, such as the discovery of Lake Sinai virus (LSV) species and the tripartite classification of deformed wing virus (DWV) associated with CCD (Runckel et al., 2011; Roberts et al., 2018a; Bonilla-Rosso et al., 2020; Daughenbaugh et al., 2021; Kadlečková et al., 2022; Li et al., 2022). Since then, several researchers have conducted virome analyses, confirming that LSV group is a virus complex with multiple species (Šimenc et al., 2020; Daughenbaugh et al., 2021; Čukanová et al., 2022; Kadlečková et al., 2022). Research has also demonstrated that DWV, which is associated with CCD, can be classified into three types (Mordecai et al., 2016; Natsopoulou et al., 2017). In some instances, plant viruses have been detected in honey bees (Granberg et al., 2013; Li et al., 2014). Interestingly, virome studies have also identified plant viruses in bees, suggesting honey bees may play a role in plant virus transmission during pollination (Darzi et al., 2018; Roberts et al., 2018b).
This study aims to explore the viral landscape within A. mellifera and A. cerana populations in Pakistan and Bangladesh. By employing virome analysis through HTS, we seek to identify the diversity of viruses present, including novel species, and provide a foundation for future virological research and conservation efforts in these regions.
MATERIALS AND METHODS
1. Honey bee sample collection
Honey bee samples were collected from multiple apiaries across Pakistan and Bangladesh (Fig. 1). In Pakistan, honey bees were obtained from the regions of Fateh Jang (33°33ʹ59.6ʺN 72°36ʹ51.1ʺE; 33°35ʹ28.9ʺN 72°40ʹ48.2ʺE), Jand (33°25ʹ57.1ʺN 72°00ʹ57.1ʺE; 33°25ʹ44.0ʺN 72°01ʹ40.2ʺE; 33°26ʹ12.8ʺN 72°00ʹ32.4ʺE), Khunda (33°27ʹ43.8ʺN 72°23ʹ55.4ʺE; 33°27ʹ31.8ʺN 72°23ʹ49.3ʺE), and PindiGheb (33°14ʹ58.1ʺN 72°16ʹ19.4ʺE; 33°14ʹ26.8ʺN 72°16ʹ20.2ʺE; 33°13ʹ56.5ʺN 72°16ʹ36.0ʺE). In Bangladesh, samples were collected from Tangail (24°14ʹ59.4ʺN 89°54ʹ59.6ʺE). From each location, a minimum of 50 honey bees were collected, preserved in 70% ethanol within 50 mL conical tubes, and stored at 4℃ until RNA extraction.
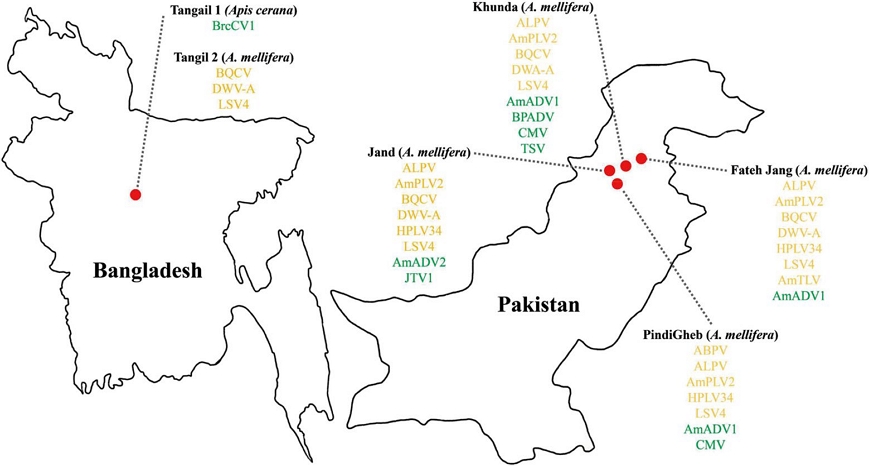
Geographic distribution of viruses identified in honey bee samples from Bangladesh and Pakistan. Red circles indicate sampling locations on the map. The list of detected viral taxa from virome analysis is shown beside each country. Viruses are color-coded based on host specificity: green for plant viruses and yellow for honey bee viruses.
2. Total RNA extraction
Ten honey bees from each sample were air-dried with tissue paper to remove residual ethanol and ground into a fine powder using a sterile pestle and mortar. Total RNA extraction was carried out using the TRIzol® reagent (Thermo Fisher Scientific, Waltham, MA, USA) following the manufacturer’s protocol. RNA concentration and purity were measured using a NanoPhotometer® NP80 (IMPLEN, Munich, Germany), and samples were subsequently stored at -80℃ until further processing for high-throughput sequencing.
3. High-throughput sequencing
For Pakistan samples, total RNA was pooled across collection sites before library preparation. Quality control of RNA was confirmed using the Bioanalyzer 2100 (Agilent Technologies, Santa Clara, CA, USA). Libraries were then constructed using the TruSeq Stranded Total RNA with Ribo-Zero H/M/R Gold kit (Illumina, San Diego, CA, USA) according to the manufacturer’s instructions. Library quality was verified again on Bioanalyzer 2100. High-throughput sequencing was performed using the Novaseq 6000 platform (Illumina) to generate raw FASTQ files.
4. Virome analysis
Raw FASTQ files were processed using the CLC Genomics Workbench (version 23.0.2, QIAGEN, Hilden, Germany). Low-quality sequences, chimeric reads, and adaptor sequence were trimmed to ensure data quality. Host-related reads were removed by mapping against Apis mellifera and A. cerana reference genomes downloaded from NCBI (NCBI, 2023a, 2023b) with sequences displaying ≥80% similarity excluded. Viral reads were identified by mapping against the NCBI viral genome database (NCBI, 2022), retaining sequences with ≥80% similarity.
To identify potential novel viruses and mutations, de novo assembly was performed on the host-filtered reads, generating contigs for further analysis. Each contig was compared against viral databases using tBLASTx, selecting sequences with E-values ≤1e-10. Confirmed viral contigs were further validated using BLASTn to refine virus identification. For suspected novel viruses with low similarity to known sequences, the assembled contigs were used directly as references to confirm complete viral genomes.
5. Phylogenetic analysis
Phylogenetic relationships of detected viruses were analyzed using IQ-TREE (Minh et al., 2020). Viral sequences, including complete viral genomes from global isolates in the NCBI database, were aligned, and a phylogenetic tree was constructed using the maximum likelihood method with 1,000 bootstrap replicates under the automatically selected best model.
RESULTS
1. High-throughput sequencing overview
The average number of reads generated per sample was 64,236,804. Notably, the Fateh Jang sample had approximately 10 million fewer reads than other samples, though all samples maintained significant Q30 values, ensuring high data quality. Tangail 1 had the highest host reads (55,812,561), while Tangail 2 held the highest viral reads count, with 23,491,534 reads (Fig. 2, Table S1). Thirteen viruses spanning seven families, along with an unclassified DNA virus, were identified (Fig. 3). Among the sixteen identified viruses, nine were honey bee viruses, while seven were plant viruses, including four novel viruses (Table 1). Apis mellifera filamentous virus (AmFV) showed the highest viral read count in Tangail 2, with approximately 20 million reads. Khunda recorded the highest virus diversity, with eight viruses identified. Plant viruses were detected in most samples, except for Fateh Jang and Tangail 2.
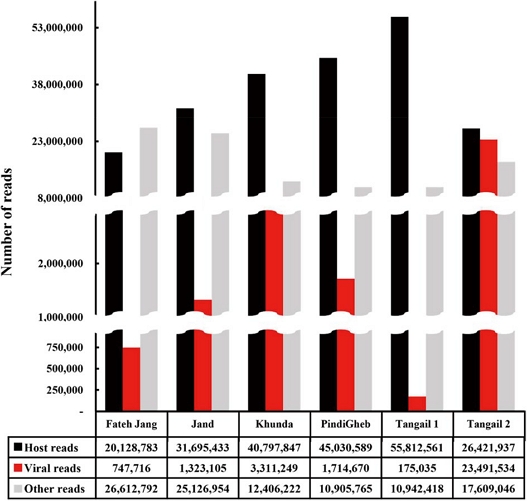
Distribution of reads following quality trimming for high-throughput sequencing. Black bars represent host-related reads, red bars represent viral reads, and gray bars represent other reads. Samples from Fateh Jang, Jand, Khunda, PindiGheb, and Tangail 1 correspond to Apis mellifera, while the Tangail 1 sample represents A. cerana.
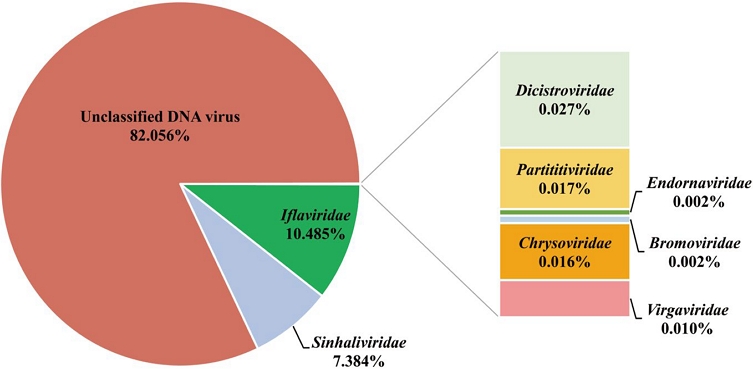
Virus read counts by family across detected viral sequences. This analysis identified eight well-known viral families and one unclassified DNA virus family from the honey bee virome samples.
2. Honey bee viruses
Aphid latent paralysis virus (ALPV) sequences from PindiGheb (PP971556) had 96.98% similarity with MF458892 from Kenya, while sequences from Fateh Jang (PP971553) and Jand (PP971554) showed 98.51% and 97.26% similarity with MW063131 from South Africa (Fig. S1A). Acute bee paralysis virus (ABPV) identified in PindiGheb (PP971549) showed 94.52% similarity with MZ821785 reported in China (Fig. S1B). Black queen cell virus (BQCV) was detected in four samples, with highest homology values of 91.88% (Fateh Jang, PP971562), 93.54% (Jand, PP971563), 93.88% (Tangail 2, PP971589), and 95% (Khunda, PP971564) to MW397638 identified in Israel (Fig. S1D). A partial sequence from the Triatovirus genus was identified in Fateh Jang (PP971572), showing 87% coverage and 80.64% identity to Vespula vulgaris picorna-like virus 2 (VvPLV2, QZZ63316) and was classified as a novel virus named “Apis mellifera associated triato-like virus (AmTLV)” (Table 2).
The Hubei partiti-like virus 34 (HPLV34) virus detected in PindiGheb (PP971577) showed 95.34% similarity to ON648754 from Slovenia, while sequences from Fateh Jang (PP971576) and Jand (PP971578) showed similarities of 95.48% and 97.63% to MT747982 from Italy (Fig. S1C). The Apis mellifera associated partiti-like virus 2 (AmPLV2), previously reported in South Korea, was detected in Pakistani samples, with sequences from Fateh Jang (PP971557) and PindiGheb (PP971559) showing 96.53% and 96.39% similarity to OR496402, and sequences from Jand (PP971558) and Khunda (PP971560) showing 96.61% and 96.24% similarity to OR496403.
Deformed wing virus (DWV) was identified in Fateh Jang (PP971573), and Tangail 2 (PP971590), showing 92.76%, 92.85%, and 93.39% similarity with MT940255 from the USA. The sequence in Jand (PP971574) exhibited 96.09% similarity with MN607197 from Viet Nam (Fig. S1E).
Lake Sinai virus 4 (LSV4) was detected in all samples except Tangail 1. The sequences showed similarities of 90.05% (Khunda, PP971580), 90.12% (Jand, PP971579), 89.30% (PindiGheb, PP971581), and 89.22% (Tangail 2, PP971591) with MZ821913 from China. The Fateh Jang (PP971582) sample yielded a partial sequence overlapping the ORF1 and RdRp regions, showing 98% similarity with LSV4 (Fig. S1F).
The complete genome sequence of Apis mellifera filamentous virus (AmFV) was confirmed in Tangail 2 from Bangladesh (PP971592). This is the fourth country where AmFV has been fully sequenced, following China (OK 392616), Hungary (OR644609-OR644611, OR270109), and Switzerland (NC_027925).
3. Plant viruses
Bell pepper alphaendornavirus (BPADV) was identified in Khunda (PP971561), showing 97.66% similarity to MH182675 from Capsicum annuum in China (Fig. S1M). Four novel virus sequences were detected, with two classified under the Alphaendornavirus genus. Alphaendornavirus 1 (provisional) showed 65-70% identity and 3-5% coverage with known sequences, while alphaendornavirus 2 (provisional) had 70% identity and 15-18% coverage (Table 2). These novel viruses, with 40% amino acid sequence similarity to previously reported viruses, were named “Apis mellifera associated alphaendornavirus 1 (AmADV1)” and “Apis mellifera associated alphaendornavirus 2 (AmADV2)”, and their sequences were uploaded to NCBI GenBank (AmADV1; PP 971550, PP971551, AmADV2; PP971552) (Fig. S1O).
Three genome segments of cucumber mosaic virus (CMV) were identified in both the Khunda and PindiGheb samples. For segment RNA1, the sequence identified in Khunda (PP971565) showed a 99.41% similarity to OQ993352, previously reported in Cucumis sativus from Canada, while the sequence from PindiGheb (PP971566) showed a 99.50% similarity to OP722596, identified in Pelargonium peltatum in Germany (Fig. S1G). Segment RNA2 showed similarities of 98.72% and 99.31% with MG882751, reported in Cucurbita pepo from Poland, in Khunda (PP971567) and PindiGheb (PP971568), respectively (Fig. S1H). For segment RNA3, the sequence identified in Khunda (PP971569) had 98.01% similarity to OQ993354 from C. sativus in Canada, and the sequence from PindiGheb (PP971570) had 98.60% similarity to ON13912 identified in Solanum lycopersicum in Greece (Fig. S1I).
Tobacco streak virus (TSV) was found in Khunda, with three RNA segments. RNA1 (PP971583) (Fig. S1J), RNA2 (PP971584) (Fig. S1K), and RNA3 (PP971585) (Fig. S1L) showed similarities of 98.72%, 99.52%, and 99.55% with Indian isolates from Gossypium barbadense, Parthenium hysterophorus, and Gomphrena globosa, respectively.
Brassica campestris chrysovirus 1 (BrcCV1), previously reported only in China, was identified in Tangail 1. Complete genome sequences of RNA1 (PP971586), RNA2 (PP971587), and RNA3 (PP971588) showed similarities of 97.3%, 98.08%, and 98.43% with NC_043661, NC_043660, and NC_043659, respectively.
One of the four novel virus sequences was classified under the Tobamovirus genus. Comparative analysis of the complete genome nucleotide sequence using BLASTn, according to ICTV genus demarcation criteria, showed 80-99% identity with previously reported tobamovirus sequences, though the coverage was limited to 1-2%. Despite the low coverage, this virus sequence contained all five conserved domains characteristic of the Tobamovirus genus (Fig. 4). Each domain showed approximately 50% similarity to known tobamovirus sequences in BLASTp analysis (Table 2). Based on these findings, this novel virus was classified as a new member of the Tobamovirus genus and named “Jand tobamovirus 1 (JTV1).” Its sequence has been deposited in NCBI GenBank (PP 971571) (Fig. S1N).

Genomic structure of Jand tobamovirus 1 (JTV1), showing the open reading frames (ORFs) and corresponding functional domains. Domains were annotated based on nucleotide positions, including Mtr (Methyltransferase), Hel (Helicase), RdRp (RNA-dependent RNA polymerase), MP (Movement protein), and CP (Coat protein).
DISCUSSION
Since the emergence of CCD in 2007, interest in honey bee viruses has surged, driving research efforts worldwide (Beaurepaire et al., 2020). HTS-based virome analyses have been conducted in many regions, revealing diverse viral profiles across Europe, North America, South America, Africa, the Middle East, and Asia (Granberg et al., 2013; Tozkar et al., 2015; Remnant et al., 2017; Galbraith et al., 2018; Haddad et al., 2018; Regan et al., 2018; Roberts et al., 2018a; Thaduri et al., 2018; Kraberger et al., 2019; Bonilla-Rosso et al., 2020; Bovo et al., 2020; Deboutte et al., 2020; Gebremedhn et al., 2020; Roberts et al., 2020; Šimenc et al., 2020; Daughenbaugh et al., 2021; da Silva et al., 2023; Kwon et al., 2023b; Lee et al., 2023; Li et al., 2023; Shojaei et al., 2023). Our study represents the first comprehensive virome analysis of two honey bee species, Apis mellifera and A. cerana, from Pakistan and Bangladesh, contributing valuable data on honey bee viruses in these countries on the Indian subcontinent.
Our results revealed the presence of multiple honey bee and plant viruses, including several previously unreported in these countries. Although the sample size is limited and may not capture the full viral diversity across all seasons and regions, this study lays the groundwork for future research. Broader, seasonal, and geographically extensive sampling is expected to reveal a more comprehensive viral landscape, potentially aiding in predicting and managing future honey bee health challenges in these regions.
Notably, we identified Apis mellifera-associated partitivirus 2 (AmPLV2), previously reported only in South Korea (Kwon et al., unpublished data, NCBI GenBank accession OR496401-OR496403), in honey bees from Pakistan. Future research should investigate the routes of transmission of this virus and its potential impact on bee health. Two novel viruses within the Alphaendornavirus genus were also identified: AmADV1 in Jand, Khunda, and PindiGheb, and AmADV2 in Jand. Given that alphaendornaviruses are pollen-transmissible (Moriyama et al., 1996), it is possible that these viruses were introduced through pollen carried by the bees. This finding underscores the potential role of honey bees as vectors for plant viruses, though it remains challenging to determine the exact plant host without further analysis.
We also detected a novel tobamovirus in honey bees from Jand. The limited sequence similarity between this virus and previously reported tobamoviruses suggests an unexplored diversity of viruses affecting plants in Pakistan (Dombrovsky et al., 2017; Zhang et al., 2022; Spiegelman and Dinesh-Kumar, 2023). Expanding virome studies to include plant hosts in Pakistan could yield new insights into viral diversity and potential reservoirs.
The viral profiles of the two bee species from Tangail, Bangladesh, showed notable differences. In A. cerana, only one plant virus was detected, while A. mellifera harbored four honey bee viruses but no plant viruses. This study also marks the first identification of Apis mellifera filamentous virus (AmFV) in A. mellifera samples from Bangladesh. Although AmFV has been linked to DWV, SBV, and BQCV in other studies (Hartmann et al., 2015), our findings provide limited data to establish relationships among these viruses. Further investigation is warranted to explore possible interactions among these pathogens.
In conclusion, this study presents the first virome analysis of honey bees in Pakistan and Bangladesh, identifying both known and novel viruses that may influence honey bee health. These findings provide foundational data for future research on honey bee viruses in these countries.
Acknowledgments
This study was conducted as part of the “Glocal University Project Group in Andong National University-Gyeongbuk Provincial College” supported by the Ministry of Education and National Research Foundation of Korea.
AUTHOR CONTRIBUTIONS
E-JK and CJ conceived and designed the study. MFK, MN-u-A prepared the samples. MK performed the experiments. E-JK and MK conducted bioinformatics and data analyses. MK and E-JK wrote the manuscript. All authors contributed to manuscript revision and have read and approved the submitted version.
References
-
Allen, M. and B. Ball. 1996. The incidence and world distribution of honey bee viruses. Bee World 77(3): 141-162.
[https://doi.org/10.1080/0005772X.1996.11099306]
- Atkins, E. 1992. Injury to honey bee poisoning. pp. 1153-1208. in The Hive and the Honey Bee, ed. by J. M. Graham. Dadant and Sons, Hamilton.
-
Bailey, L., B. V. Ball and J. Perry. 1983. Association of viruses with two protozoal pathogens of the honey bee. Ann. Appl. Biol. 103(1): 13-20.
[https://doi.org/10.1111/j.1744-7348.1983.tb02735.x]
-
Beaurepaire, A., N. Piot, V. Doublet, K. Antunez, E. Campbell, P. Chantawannakul, N. Chejanovsky, A. Gajda, M. Heerman and D. Panziera. 2020. Diversity and global distribution of viruses of the western honey bee, Apis mellifera. Insects 11(4): 239.
[https://doi.org/10.3390/insects11040239]
-
Bonilla-Rosso, G., T. Steiner, F. Wichmann, E. Bexkens and P. Engel. 2020. Honey bees harbor a diverse gut virome - engaging in nested strain-level interactions with the microbiota. Proc. Natl. Acad. Sci. 117(13): 7355-7362.
[https://doi.org/10.1073/pnas.2000228117]
-
Bovo, S., V. J. Utzeri, A. Ribani, R. Cabbri and L. Fontanesi. 2020. Shotgun sequencing of honey DNA can describe honey bee derived environmental signatures and the honey bee hologenome complexity. Sci. Rep. 10(1): 9279.
[https://doi.org/10.1038/s41598-020-66127-1]
-
Chen, Y. P. and R. Siede. 2007. Honey bee viruses. Adv. Virus Res. 70: 33-80.
[https://doi.org/10.1016/S0065-3527(07)70002-7]
-
Choe, S. E., L. T. Nguyen, J. H. Noh, C. H. Kweon, K. E. Reddy, H. B. Koh, K. Y. Chang and S. W. Kang. 2012. Analysis of the complete genome sequence of two Korean sacbrood viruses in the Honey bee, Apis mellifera. Virology 432(1): 155-161.
[https://doi.org/10.1016/j.virol.2012.06.008]
-
Collins, F. S., M. Morgan and A. Patrinos. 2003. The Human Genome Project: lessons from large-scale biology. Science 300(5617): 286-290.
[https://doi.org/10.1126/science.1084564]
-
Conesa, A., P. Madrigal, S. Tarazona, D. Gomez-Cabrero, A. Cervera, A. McPherson, M. W. Szcześniak, D. J. Gaffney, L. L. Elo and X. Zhang. 2016. A survey of best practices for RNA-seq data analysis. Genome Biol. 17(1): 1-19.
[https://doi.org/10.1186/s13059-016-0881-8]
-
Čukanová, E., R. Moutelíková and J. Prodělalová. 2022. First detection of Lake Sinai virus in the Czech Republic: a potential member of a new species. Arch. Virol. 167(11): 2213-2222.
[https://doi.org/10.1007/s00705-022-05548-x]
-
da Silva, L. A., B. R. de Camargo, B. M. P. Rodrigues, D. L. Berlitz, L. M. Fiuza, D. M. P. Ardisson-Araújo and B. M. Ribeiro. 2023. Exploring viral infections in honey bee colonies: insights from a metagenomic study in southern Brazil. Brazi. J. Microbiol. 54(3): 1447-1458.
[https://doi.org/10.1007/s42770-023-01078-z]
-
Darzi, E., E. Smith, D. Shargil, O. Lachman, L. Ganot and A. Dombrovsky. 2018. The honeybee Apis mellifera contributes to Cucumber green mottle mosaic virus spread via pollination. Plant Pathol. 67(1): 244-251.
[https://doi.org/10.1111/ppa.12702]
-
Daughenbaugh, K. F., I. Kahnonitch, C. C. Carey, A. J. McMenamin, T. Wiegand, T. Erez, N. Arkin, B. Ross, B. Wiedenheft and A. Sadeh. 2021. Metatranscriptome analysis of sympatric bee species identifies bee virus variants and a new virus, Andrena-associated bee virus-1. Viruses 13(2): 291.
[https://doi.org/10.3390/v13020291]
-
Deamer, D., M. Akeson and D. Branton. 2016. Three decades of nanopore sequencing. Nat. Biotechnol. 34(5): 518-524.
[https://doi.org/10.1038/nbt.3423]
-
Deboutte, W., L. Beller, C. K. Yinda, P. Maes, D. C. de Graaf and J. Matthijnssens. 2020. Honey-bee-associated prokaryotic viral communities reveal wide viral diversity and a profound metabolic coding potential. Proc. Natl. Acad. Sci. 117(19): 10511-10519.
[https://doi.org/10.1073/pnas.1921859117]
-
Dombrovsky, A., L. T. Tran-Nguyen and R. A. Jones. 2017. Cucumber green mottle mosaic virus: rapidly increasing global distribution, etiology, epidemiology, and management. Annu. Revi. Phytopathol. 55: 231-256.
[https://doi.org/10.1146/annurev-phyto-080516-035349]
-
Ercolini, D. 2013. High-throughput sequencing and metagenomics: moving forward in the culture-independent analysis of food microbial ecology. Appl. Environ. Microbiol. 79(10): 3148-3155.
[https://doi.org/10.1128/AEM.00256-13]
-
Feng, Y., Y. Zhang, C. Ying, D. Wang and C. Du. 2015. Nanopore-based fourth-generation DNA sequencing technology. Genom. Proteom. Bioinf. 13(1): 4-16.
[https://doi.org/10.1016/j.gpb.2015.01.009]
-
Galbraith, D. A., Z. L. Fuller, A. M. Ray, A. Brockmann, M. Frazier, M. W. Gikungu, J. Martinez, K. M. Kapheim, J. T. Kerby and S. D. Kocher. 2018. Investigating the viral ecology of global bee communities with high-throughput metagenomics. Sci. Rep. 8(1): 1-11.
[https://doi.org/10.1038/s41598-018-27164-z]
-
Gebremedhn, H., W. Deboutte, K. Schoonvaere, P. Demaeght, L. De Smet, B. Amssalu, J. Matthijnssens and D. C. de Graaf. 2020. Metagenomic approach with the Neto-VIR enrichment protocol reveals virus diversity within ethiopian honey bees (Apis mellifera simensis). Viruses 12(11): 1218.
[https://doi.org/10.3390/v12111218]
-
Granberg, F., M. Vicente-Rubiano, C. Rubio-Guerri, O. E. Karlsson, D. Kukielka, S. Belák and J. M. Sánchez-Vizcaíno. 2013. Metagenomic detection of viral pathogens in Spanish honeybees: co-infection by aphid lethal paralysis, Israel acute paralysis and Lake Sinai viruses. PLoS One 8(2): e57459.
[https://doi.org/10.1371/journal.pone.0057459]
-
Haddad, N., L. Horth, B. Al-Shagour, N. Adjlane and W. Loucif-Ayad. 2018. Next-generation sequence data demonstrate several pathogenic bee viruses in Middle East and African honey bee subspecies (Apis mellifera syriaca, Apis mellifera intermissa) as well as their cohabiting pathogenic mites (Varroa destructor). Virus Genes 54(5): 694-705.
[https://doi.org/10.1007/s11262-018-1593-9]
- Haque, A. 2023. In Bangladesh, there are some 12,000-15,000 such nomadic beekeepers, with three to seven employees on each farm. In search of honey: The lives of nomadic beekeepers of Bangladesh, from https://www.tbsnews.net/features/panorama/search-honey-lives-nomadic-beekeepers-bangladesh-588678, .
-
Hartmann, U., E. Forsgren, J.-D. Charrière, P. Neumann and L. Gauthier. 2015. Dynamics of Apis mellifera filamentous virus (AmFV) infections in honey bees and relationships with other parasites. Viruses 7(5): 2654-2667.
[https://doi.org/10.3390/v7052654]
-
Kadlečková, D., R. Tachezy, T. Erban, W. Deboutte, J. Nunvář, M. Saláková and J. Matthijnssens. 2022. The virome of healthy honey bee colonies: ubiquitous occurrence of known and new viruses in bee populations. mSystems 7(3): e00072-00022.
[https://doi.org/10.1128/msystems.00072-22]
-
Kapoor, A. and W. I. Lipkin. 2014. Virus discovery in the 21st century. Encyclopedia of Life Sciences.
[https://doi.org/10.1002/9780470015902.a0023621]
-
Khan, K. A. and H. A. Ghramh. 2023. Beekeeping in Pakistan: history, potential, and current status. Pak. J. Zool. 56(1): 421-428.
[https://doi.org/10.17582/journal.pjz/20230419090432]
-
Kraberger, S., C. N. Cook, K. Schmidlin, R. S. Fontenele, J. Bautista, B. Smith and A. Varsani. 2019. Diverse single-stranded DNA viruses associated with honey bees (Apis mellifera). Infect. Genet. Evol. 71: 179-188.
[https://doi.org/10.1016/j.meegid.2019.03.024]
-
Kwon, M., C. Jung and E.-J. Kil. 2023a. Honey bee viruses: an ongoing history of discovery. J. Apic. 38(2): 121-137.
[https://doi.org/10.17519/apiculture.2023.06.38.2.121]
-
Kwon, M., C. Jung and E.-J. Kil. 2023b. Metagenomic analysis of viromes in honey bee colonies (Apis mellifera; Hymenoptera: Apidae) after mass disappearance in Korea. Front. Cell. Infect. Microbiol. 13: 27.
[https://doi.org/10.3389/fcimb.2023.1124596]
-
Le Conte, Y. and M. Navajas. 2008. Climate change: impact on honey bee populations and diseases. Rev. Sci. Tech.-OIE 27(2): 499-510.
[https://doi.org/10.20506/rst.27.2.1819]
-
Lee, E., R. Vansia, J. Phelan, A. Lofano, A. Smith, A. Wang, G. J. Bilodeau, S. F. Pernal, M. M. Guarna and M. Rott. 2023. Area wide monitoring of plant and honey bee (Apis mellifera) Viruses in Blueberry (Vaccinium corymbosum) agroecosystems facilitated by honey bee pollination. Viruses 15(5): 1209.
[https://doi.org/10.3390/v15051209]
-
Li, J. L., R. S. Cornman, J. D. Evans, J. S. Pettis, Y. Zhao, C. Murphy, W. J. Peng, J. Wu, M. Hamilton and H. F. Boncristiani Jr. 2014. Systemic spread and propagation of a plant-pathogenic virus in European honeybees, Apis mellifera. MBio 5(1): e00898-00813.
[https://doi.org/10.1128/mBio.00898-13]
-
Li, N., C. Li, T. Hu, J. Li, H. Zhou, J. Ji, J. Wu, W. Kang, E. C. Holmes and W. Shi. 2023. Nationwide genomic surveillance reveals the prevalence and evolution of honeybee viruses in China. Microbiome 11(1): 6.
[https://doi.org/10.1186/s40168-022-01446-1]
-
Li, N., Y. Huang, W. Li and S. Xu. 2022. Virome analysis reveals diverse and divergent RNA viruses in wild insect pollinators in Beijing, China. Viruses 14(2): 227.
[https://doi.org/10.3390/v14020227]
-
Liu, X., Y. Zhang, X. Yan and R. Han. 2010. Prevention of Chinese sacbrood virus infection in Apis cerana using RNA interference. Curr. Microbiol. 61: 422-428.
[https://doi.org/10.1007/s00284-010-9633-2]
-
Mardis, E. R. 2011. A decade’s perspective on DNA sequencing technology. Nature 470(7333): 198-203.
[https://doi.org/10.1038/nature09796]
-
Minh, B. Q., H. A. Schmidt, O. Chernomor, D. Schrempf, M. D. Woodhams, A. Von Haeseler and R. Lanfear. 2020. IQ-TREE 2: new models and efficient methods for phylogenetic inference in the genomic era. Mol. Biol. Evol. 37(5): 1530-1534.
[https://doi.org/10.1093/molbev/msaa015]
-
Mordecai, G. J., L. Wilfert, S. J. Martin, I. M. Jones and D. C. Schroeder. 2016. Diversity in a honey bee pathogen: first report of a third master variant of the Deformed Wing Virus quasispecies. ISME J 10(5): 1264-1273.
[https://doi.org/10.1038/ismej.2015.178]
-
Moriyama, H., K. Kanaya, J. Wang, T. Nitta and T. Fukuhara. 1996. Stringently and developmentally regulated levels of a cytoplasmic double-stranded RNA and its high-efficiency transmission via egg and pollen in rice. Plant Mol. Biol. 31: 713-719.
[https://doi.org/10.1007/BF00019459]
-
Natsopoulou, M. E., D. P. McMahon, V. Doublet, E. Frey, P. Rosenkranz and R. J. Paxton. 2017. The virulent, emerging genotype B of Deformed wing virus is closely linked to overwinter honeybee worker loss. Sci. Rep. 7(1): 1-9.
[https://doi.org/10.1038/s41598-017-05596-3]
- NCBI. 2022. NCBI Virus: Sequences for discovery. Retrieved 12.20, 2022, from https://www.ncbi.nlm.nih.gov/labs/virus/vssi/#/virus?SeqType_s=Nucleotide&VirusLineage_ss=Viruses,%20taxid:10239&HostLineage_ss=Apis%20mellifera%20(honey%20bee),%20taxid:7460, .
- NCBI. 2023a. NCBI Genome Apis cerana. Retrieved 12.30, 2023, from https://www.ncbi.nlm.nih.gov/datasets/taxonomy/7461/, .
- NCBI. 2023b. NCBI Genome Apis mellifera. Retrieved 12.30, 2023, from https://www.ncbi.nlm.nih.gov/genome/?term=apis+mellifera, .
-
Nguyen, N. T. B. and T. H. Le. 2013. Complete genome sequence of sacbrood virus strain SBM2, isolated from the honeybee Apis cerana in Vietnam. Genome Announc. 1(1): e00076-12.
[https://doi.org/10.1128/genomeA.00076-12]
-
Oldroyd, B. P. 2007. What’s killing American honey bees? PLoS Biol. 5(6): e168.
[https://doi.org/10.1371/journal.pbio.0050168]
-
Pareek, C. S., R. Smoczynski and A. Tretyn. 2011. Sequencing technologies and genome sequencing. J. Appl. Genet. 52: 413-435.
[https://doi.org/10.1007/s13353-011-0057-x]
-
Popovska Stojanov, D., L. Dimitrov, J. Danihlík, A. Uzunov, M. Golubovski, S. Andonov and R. Brodschneider. 2021. Direct economic impact assessment of winter honeybee colony losses in Three European countries. Agriculture 11(5): 398.
[https://doi.org/10.3390/agriculture11050398]
-
Regan, T., M. W. Barnett, D. R. Laetsch, S. J. Bush, D. Wragg, G. E. Budge, F. Highet, B. Dainat, J. R. De Miranda and M. Watson. 2018. Characterisation of the British honey bee metagenome. Nat. Commun. 9(1): 4995.
[https://doi.org/10.1038/s41467-018-07426-0]
-
Rehman, M., S. Ahmad, M. Shah and I. Khan. 2018. Identification of varroa mite species of honeybee, Apis mellifera in Khyber Pukhtunkhawa Peshawar, Pakistan. Sarhad J. Agric. 34(2): 414-417.
[https://doi.org/10.17582/journal.sja/2018/34.2.414.417]
-
Remnant, E. J., M. Shi, G. Buchmann, T. Blacquière, E. C. Holmes, M. Beekman and A. Ashe. 2017. A diverse range of novel RNA viruses in geographically distinct honey bee populations. J. Virol. 91(16): e00158-17.
[https://doi.org/10.1128/jvi.00158-00117]
-
Rhoads, A. and K. F. Au. 2015. PacBio sequencing and its applications. Genom. Proteom. Bioinf. 13(5): 278-289.
[https://doi.org/10.1016/j.gpb.2015.08.002]
-
Roberts, J. M., D. L. Anderson and P. A. Durr. 2018a. Metagenomic analysis of Varroa-free Australian honey bees (Apis mellifera) shows a diverse Picornavirales virome. J. Gen. Virol. 99(6): 818-826.
[https://doi.org/10.1099/jgv.0.001073]
-
Roberts, J. M., K. B. Ireland, W. T. Tay and D. Paini. 2018b. Honey bee-assisted surveillance for early plant virus detection. Ann. Appl. Biol. 173(3): 285-293.
[https://doi.org/10.1111/aab.12461]
-
Roberts, J. M., N. Simbiken, C. Dale, J. Armstrong and D. L. Anderson. 2020. Tolerance of honey bees to Varroa mite in the absence of deformed wing virus. Viruses 12(5): 575.
[https://doi.org/10.3390/v12050575]
-
Runckel, C., M. L. Flenniken, J. C. Engel, J. G. Ruby, D. Ganem, R. Andino and J. L. DeRisi. 2011. Temporal analysis of the honey bee microbiome reveals four novel viruses and seasonal prevalence of known viruses, Nosema, and Crithidia. PLoS One 6(6): e20656.
[https://doi.org/10.1371/journal.pone.0020656]
-
Schloss, J. A. 2008. How to get genomes at one ten-thousandth the cost. Nat. Biotechnol. 26(10): 1113-1115.
[https://doi.org/10.1038/nbt1008-1113]
-
Sekse, C., A. Holst-Jensen, U. Dobrindt, G. S. Johannessen, W. Li, B. Spilsberg and J. Shi. 2017. High throughput sequencing for detection of foodborne pathogens. Front. Microbiol. 8: 2029.
[https://doi.org/10.3389/fmicb.2017.02029]
-
Shojaei, A., A. Nourian, M. Khanjani and P. Mahmoodi. 2023. The first molecular characterization of Lake Sinai virus in honey bees (Apis mellifera) and Varroa destructor mites in Iran. J Apic. Res. 62(5): 1176-1182.
[https://doi.org/10.1080/00218839.2021.1921467]
-
Šimenc, L., U. Kuhar, U. Jamnikar-Ciglenečki and I. Toplak. 2020. First complete genome of Lake Sinai Virus Lineage 3 and genetic diversity of Lake Sinai virus Strains from honey bees and bumble bees. J. Econ. Entomol. 113(3): 1055-1061.
[https://doi.org/10.1093/jee/toaa049]
-
Spiegelman, Z. and S. P. Dinesh-Kumar. 2023. Breaking Boundaries: The Perpetual Interplay Between Tobamoviruses and Plant Immunity. Annu. Rev. Virol. 10.
[https://doi.org/10.1146/annurev-virology-111821-122847]
- TBS. 2020. Bees, the most important insect on earth, are dead because of indiscriminate fogging of insecticides by the city corporation to kill mosquitoes. Vanishing Bees: Our existential threat, from https://www.tbsnews.net/environment/nature/most-important-living-beings-earth-42979, .
-
Thaduri, S., B. Locke, F. Granberg and J. R. de Miranda. 2018. Temporal changes in the viromes of Swedish Varroa-resistant and Varroa-susceptible honeybee populations. PLoS One 13(12): e0206938.
[https://doi.org/10.1371/journal.pone.0206938]
-
Theisen-Jones, H. and K. Bienefeld. 2016. The Asian honey bee (Apis cerana) is significantly in decline. Bee World 93(4): 90-97.
[https://doi.org/10.1080/0005772X.2017.1284973]
-
Tozkar, C. Ö., M. Kence, A. Kence, Q. Huang and J. D. Evans. 2015. Metatranscriptomic analyses of honey bee colonies. Front. Genet. 6: 100.
[https://doi.org/10.3389/fgene.2015.00100]
-
Waghchoure-Camphor, E. S. and S. J. Martin. 2009. Population changes of Tropilaelaps clareae mites in Apis mellifera colonies in Pakistan. J. Apic. Res. 48(1): 46-49.
[https://doi.org/10.3896/IBRA.1.48.1.10]
-
White, G. F. 1913. Sacbrood, a disease of bees, US: Government Printing Office.
[https://doi.org/10.5962/bhl.title.56965]
-
Zacepins, A., A. Kviesis, V. Komasilovs and R. Brodschneider. 2021. When it pays to catch a swarm - evaluation of the economic importance of remote honey bee (Apis mellifera) colony swarming detection. Agriculture 11(10): 967.
[https://doi.org/10.3390/agriculture11100967]
-
Zhang, S., J. S. Griffiths, G. Marchand, M. A. Bernards and A. Wang. 2022. Tomato brown rugose fruit virus: an emerging and rapidly spreading plant RNA virus that threatens tomato production worldwide. Mol. Plant Pathol. 23(9): 1262-1277.
[https://doi.org/10.1111/mpp.13229]
Appendix
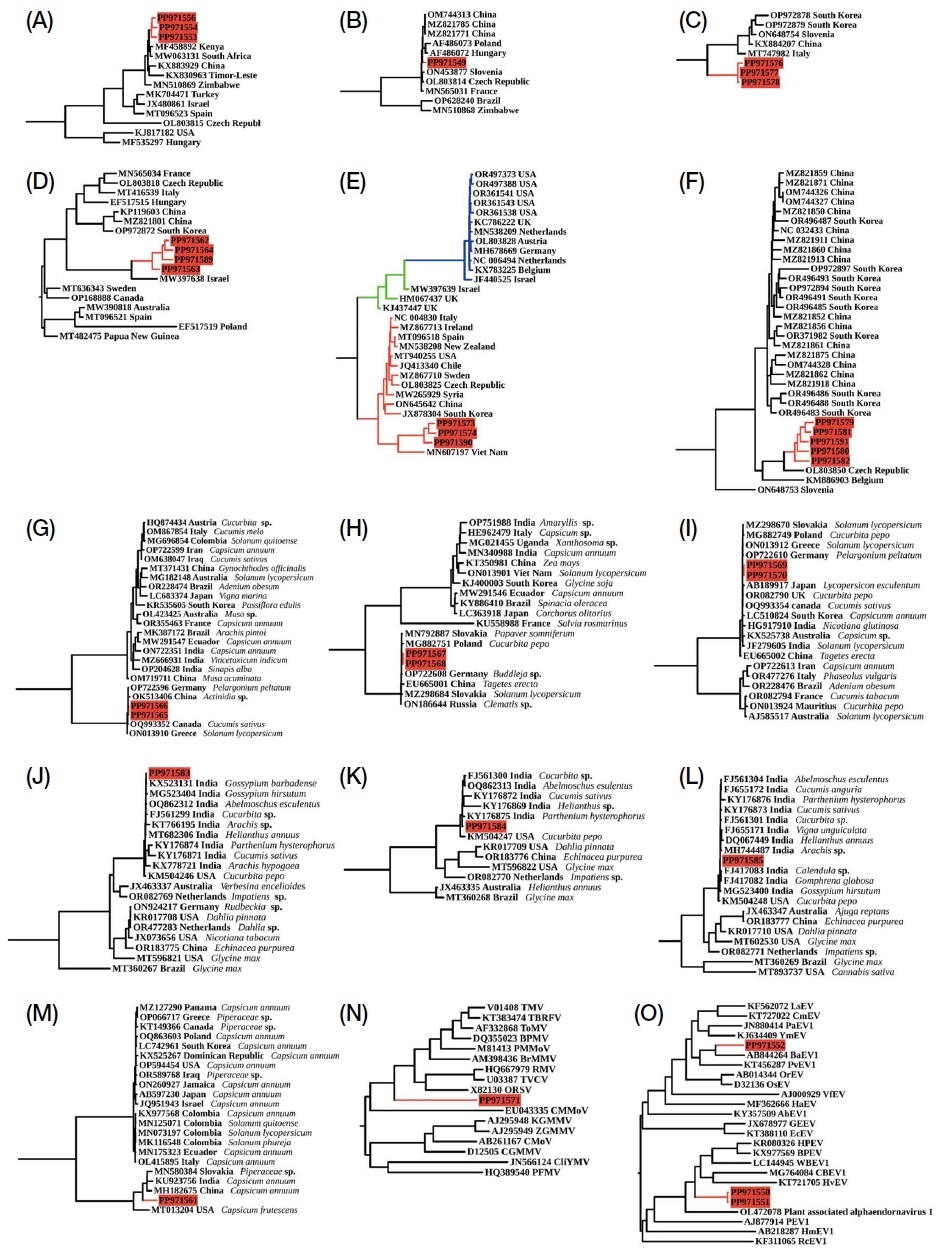
Maximum likelihood phylogenetic trees constructed using IQ-TREE2 with 1,000 bootstrap replicates. The models were selected based on the Bayesian Information Criterion (BIC): (A) Aphid latent paralysis virus (ALPV) using the TIM2+F+I+G4. (B) Acute bee paralysis virus (ABPV) using the TIM2+F+G4 model. (C) Hubei partiti-like virus 34 (HPLV34) using the HKY+F+I model. (D) Black queen cell virus (BQCV) using the GTR+F+I+G4 model. (E) Deformed wing virus (DWV) using the TIM2+F+I+G4 model. DWV-A (red line), DWV recombinant (green line), DWV-B (blue line). (F) Lake Sinai virus 4 (LSV4) using the TN+F+I+G4 model. (G) Cucumber mosaic virus (CMV) RNA1 using the GTR+F+I+G4 model. (H) CMV RNA2 using the TIM2+F+G4 model. (I) CMV RNA3 using the TIM3+F+G4 model. (J) Tobacco streak virus (TSV) RNA1 using the TVM+F+G4 model. (K) TSV RNA2 using the TIM2+F+G4 model. (L) TSV RNA3 using the TVMe+G4 model. (M) BPADV using the TIM2+F+G4 model. (N) Jand tobamovirus 1 (JTV1) using the GTR+F+I+G4 model. (O) Apis mellifera associated alphaendornavirus (AmADV) using the GTR+F+I+G4 model. Bootstrap support values are provided at key nodes, demonstrating high confidence in the tree topology. Viral sequences identified in this study are highlighted in red.