
Acute Oral Toxicity of Neonicotinoid Insecticides to Four Species of Honey Bee, Apis florea, A. cerana, A. mellifera, and A. dorsata
Abstract
Honeybees are important pollinators in agricultural and natural ecosystems. Conservation of wild pollinators as well as managed honeybees are critical for sustainable ecosystem service. Honeybees are exposed to various threats including pesticides. We tested the susceptibility of the neonicotinoids; Thiamethoxam, Imidacloprid, and Clothianidin, and a carbamate pesticide; carbaryl to four species of honey bees, Apis florea, A. cerana, A. mellifera, and A. dorsata from northern Thailand. Formulated pesticide products were serially diluted and utilized for oral toxicity test. When treated with the recommended concentrations, all tested adult bees were dead within 18 hr for all tested chemicals. Feral honeybee species showed higher LC50 values for 3 neonicotinoids than the managed honeybees. When treated with carbaryl, LC50 values were not different between the honey bee species. The results indicate that tested insecticides would pose risks not only to managed honeybees but also the indigenous honey bee species. Additionally Korean populations of A. mellifera showed higher LC50 values for 4 pesticides compared to the Thailand A. mellifera population, indicating that there might be complex interactions of differential exposures of those chemicals in agricultural fields and honeybees.
Keywords:
LC50, Feral honeybee, Apis florea, A. cerana, A. mellifera, A. dorsataINTRODUCTION
Honeybees are known as economically important social insects and provide various products such as honey, royal jelly, propolis, bee pollen and bee venom in a variety of uses. They are also important pollinators in agricultural and natural landscape ecosystems. Honeybees, primarily Apis mellifera, remain the most economically important pollinator in crop species (McGregor, 1976; Watanabe, 1994) dependent on insect pollination for their production. In the world, the majority of crops (ca. 71%) are beepollinated, accounting for 90% of food supply for 146 countries (Klein et al., 2007). Economic contribution of pollination to crop production was estimated to be 2-7 trillion USD worldwide and over 6 billion USD in Korea (Jung, 2008). However, there are increasing concerns regarding pollinator decline including honeybees. Pollinator populations are declining in many parts of the world (Lebuhn et al., 2012). Especially, the putative decline of the European honeybee, A. mellifera has been reported in developed counties such as USA, Europe, Australia and New Zealand, even though the decline is not a global unidirectional trend (Aizen et al., 2009). Because such a large portion of the human food supply is derived from pollinator dependent crops, honeybee decline implies a risk of reduced food production, the so called pollination deficit from pollinator deficit (Kevan, 1999).
Recently habitat deterioration including habitat degradation and fragmentation of natural landscapes (Thomas et al., 2004), higher pathogen prevalence (Colla et al., 2006; Cordes et al., 2012; Graystock et al., 2013; Furst et al., 2014), competition between native and invasive species (Goulson, 2003), climate change and agricultural intensification have led to less plant diversity as well as increased usage of chemical inputs such as fertilizer and pesticides (Atkins, 1992; Kluser et al., 2007). The consequences of pesticides on pollinators are well documented and understood (Johansen and Mayer, 1990; Sihag, 1995). The non-target effects of neonicotinoid insecticides has been well established and some of these insecticides are already banned for commercial uses in Europe (EU, 2013). In Korea, beekeepers have historically experienced honeybee poisoning by such standard insecticides such as carbaryl (Kim and Jung, 2013b).
A. mellifera has been in the spotlight of attention since the detection of CCD (Colony Collapse Disorder) which resulted in the initiation of multiple conservation efforts. However, information on the Asian species of honeybees relative to the negative impacts of pesticides is limited, even though many Asian honeybee populations are in state of extinction risk such as the indigenous Apis cerana population in Korea (Jung and Cho, 2015). In many parts of Asia, A. florea, A. cerana, and A. dorsata are exploited by humans for honey harvesting as well as for crop pollination (Oldroyd and Wongsiri, 2006). In several Asian countries, European honeybees are well established and function as the primary bee species for honey production (Jung and Cho, 2015) and managed pollination service.
Neonicotinoids are neuro-active insecticides chemically similar to nicotine. Neonicotinoids bind to nicotinic acetylcholine receptors of a cell and triggers a response by that cell. Nicotinic acetylcholine receptors are activated by the neurotransmitter acetylcholine. While low to moderate activation causes nervous stimulation, high levels over stimulate and block the receptors (Yamamoto, 1999) causing paralysis and death. Acetylcholinesterase breaks down acetylcholine to terminate signals from these receptors. However, acetylcholin esterase cannot break down neonicotinoids and their binding is irreversible. Compared to organophosphate and carbamate insecticides, neonicotinoids cause less toxicity in birds and mammals. However, neonicotinoid use has been linked in a range of studies to adverse ecological effects, including honeybee colony collapse disorder (CCD) and loss of birds due to the reduction in insect populations. In 2013, the European Union and a few non-EU countries restricted the use of certain neonicotinioids (Cressey, 2013). Even with the increasing concerns on the toxicity of neonicotinoid pesticides, those are still the most prevailing group of pesticides to control various agricultural arthropod pests. In this study, we compared the toxicity of three neonicotinoid insecticides and one carbamate to four species of honeybees relative to the body size. We also compared the toxicological data of A. mellifera from two geographic locations where the use of agricultural inputs are significantly different; Chiang Mai, Thailand and Andong, Korea which could result in evaluating the possibility for using honeybees as bio-indicators to monitor environmental stresses brought by biotic and abiotic factors.
MATERIALS AND METHODS
Test species
Honeybee toxicity tests were conducted at Chiang Mai University (CMU) in northern Thailand in mid January 2015. The tests were carried out on four species of honeybees; Apis mellifera L., A. cerana F., (domesticated population), Apis florea F., and A. dorsata F., (feral populations). The feral populations of A. florea and A. dorsata were collected in situ from the CMU campus. Adult bee workers were placed in refrigeration (4°C) shortly following collection. After cooling, individual bees were transferred into the test system the same day as collection. Experimental populations of A. cerana and A. were obtained from the CMU experimental apiary. For A. florea and A. dorsata mixed ages of workers bees were used in the testing. However, for the managed honey bees, A. cerana and mellifera, young house-keeping bees were selected for the tests. Body size and body mass were estimated in mm and mg scales, respectively (Fig. 1). Apis florea is the smallest and A. dorsata is the largest (Oldroyd and Wongsiri, 2006). Differences of body length and mass was ca. 2 and 5 times respectively.
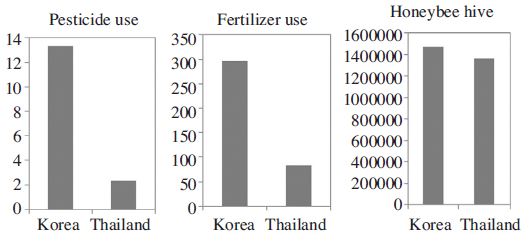
Environmental indicators, pesticide use (kg/ha, average of 1992-2010) and fertilizer use (Kg/ha, average of 2002-2010) of agro-ecosystems in the Republic of Korea and Thailand (Source: FAOSTAT).
Pesticides and test system
Three neonicotinoid insecticides (thiamethoxam, imidacloprid, clothianidin) and one carbamate (carbaryl) were used for the acute oral toxicity tests of four species of honeybees. Commercial formulations available in Korea were used for simulating the actual field situation where formulated insecticides would be applied (Table 1). Individual bees were used in the tests. Individual honey bees were exposed to 1.5ml of 30% sugar solution in which different concentrations of pesticides were added (n=30 or 50). Sugar solution (Pls provide concentration ratio of water and sugar) was provided in a 1.5ml tube inside a 50ml transparent plexiglass cup on which several holes were provided for ventilation. Pesticide concentrations were prepared from recommended concentration to the lower level until 10-5 (Table 2). In the control treatment, only sugar solution was provided without any insecticides. After exposure of the individuals to the pesticide contaminated sugar solution, mortality was checked at 1, 4, 8, 12, 24 hours after treatment. If there is no movement of appendages when touched by a hair brush, then the test bee was considered dead.
Additionally, the toxicological data for A. mellifera from two geographic locations were compared. For that the same experimental set-up as used in the Thailand study were repeated in Andong, Korea using a Korean population of A. mellifera in mid-May, 2015. For the two countries, we collected pesticide and fertilizer use data from the FAO database (FAOSTAT, access at 2015) for the past 20 years, and Korean honeybee hive numbers from FAOSTAT, and for Thailand from Oldroyd and Wongsiri (2006).
Data analysis
Dose-mortality relationships were analyzed based on the treatment concentration rather than treatment dose. In our experiments, it was not possible to measure the amount of oral uptake of the treated pesticide-contaminated sugar solution per each individual bee. Thus, median lethal concentration (LC50) and median lethal time (LT50) were estimated by probit analysis using Polo Plus program (LeOra software, 2007). T-tests were conducted to compare the differences between the toxicity at the two locations, Andong Korea and Chiang Mai Thailand.
RESULT
Toxicity relative to honeybee species
There were significant differences observed in the LD50 for different pesticides (Pls provide stat values) and also among different honeybee species (Pls provide stat values) (Table 2). However, a consistent size effect was not found among tested chemicals. Thiamethoxam LC50 values at 12 hours after treatment (HAT) were low for A. cerana and A. mellifera and high for A. florea and A. dorsata. A similar pattern was found for imidacloprid. Thiamethoxam LC50 values at 12 HAT showed the lowest value for A. mellifera and highest for A. dorsata. For carbaryl, there was no difference in LC50 values for all species tested. Among the tested chemicals, LC50 values of carbaryl were the highest.
Mortality from thiamethoxam intake occurred earlier than other pesticides (3.2, <1, <1, and 1.5 HAT for A. florea, A. cerana, A. mellifera, and A. dorsata respectively). Median lethal times at recommended concentrations of each chemical for A. cerana were all less than 1 hr. Mortality times lengthened for A. florea and A. dorsata (Table 3).
Toxicity relative to A. mellifera from two locations
When comparing the toxicity data from two locations, the Andong A. mellifera population showed much higher LC50 values than Chiang Mai population for all insecticides from 5 times (carbaryl), 40 times (clothianidin) to 100 times (imidacloprid and thiamethoxam) (Table 4). Pesticide and fertilizer use (kg/ha) was 3-6 times higher in Korea as compared to Thailand (Fig. 1).
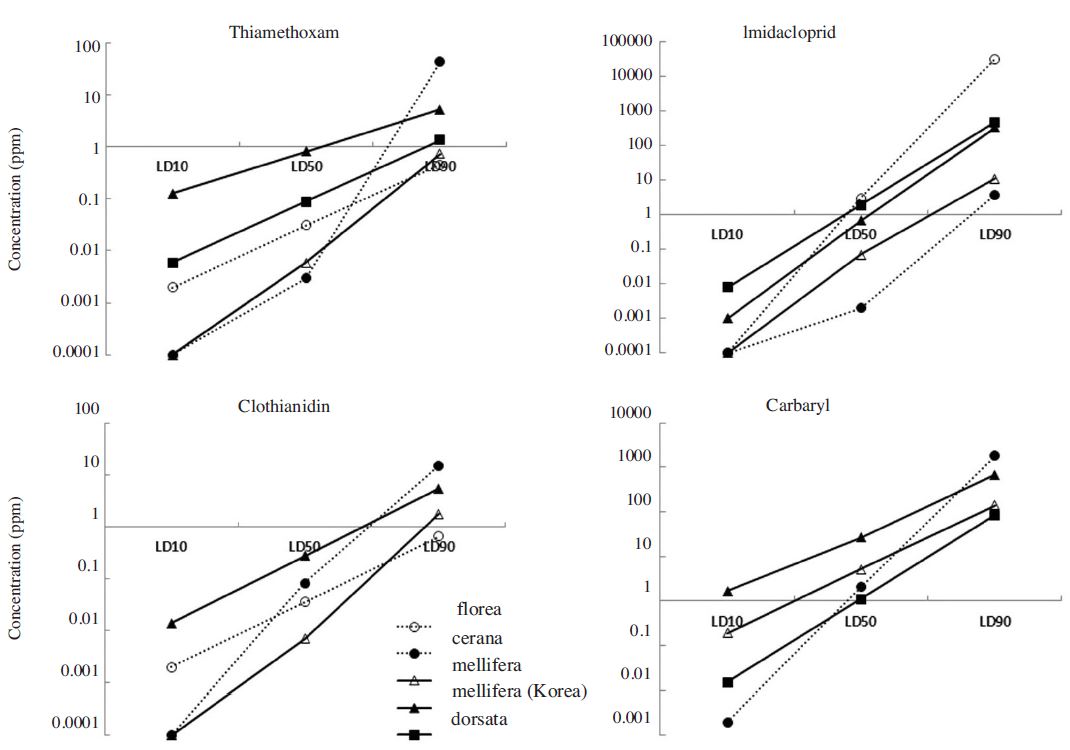
LC10, 50, and 90 values of 4 insecticides tested for four species of honey bees; Apis florea, A. cerana, A. mellifera, A. dorsata from Chiang Mai, Thailand and A. mellifera of Andong Korea.
DISCUSSION
This study clearly demonstrates that the tested pesticides are harmful not only to managed Apis mellifera and A. cerana but also the feral honeybee species A. florea and A. dorsata. Even though the distribution of the feral honeybees could be more remote or separated from major agricultural systems, chances for hazardous pesticide exposure exist for feral honeybees (Oldroyd and Wongsiri, 2006). Usually the median lethal dose or median lethal concentration can be used to evaluate the possible pesticide risk to beneficial organisms. Surprisingly LC50 patterns to the tested neonicotinoids were in contrast to our expectation of higher sensitivity for the smaller honey bee, A. florea. From the results, managed honey bees, A. mellifera and A. cerana were more sensitive and resulted in lower LC50 values. Higher LC50 values for A. florea and A. dorsata were observed. The difference of toxicity patterns found could have behavioral or physiological explanations. Managed species of A. mellifera and A. cerana could have taken up larger amounts of the contaminated sugar solution even under the disturbed experimental conditions, partly because they would have experienced the sugar solution which beekeepers had provided during dearth periods (Roubik and Buchmann, 1984; Scheiner et al., 2003), and partly because these managed honeybees often experience repeated disturbance of their bee hive by beekeepers. On the contrary, feral bees would not take up the pesticide-contaminated sugar solution as readily possibly because of the disturbance or enhanced ability to sense the exotic chemicals. Another possibility comes from the physiological mechanism of detoxification of the pesticide. Du Rand et al. (2015) showed that using nicotine alternatives to neonicotinoids, honeybees showed active detoxification of nicotine associated with increased energetic investment and also antioxidant and heat shock responses. The increased energetic investment is significant in view of the interactions of pesticides with diseases such as Nosema spp. which cause energetic stress and possible malnutrition.
LC50 values of carbaryl were not different among tested honey bee species. Carbaryl, 1-naphthyl methylcarbamate, is the carbamate insecticide which had been widely used for insect control since 1950s when it was introduced for agricultural pest insect management (Claudianos et al., 2006). Thus the bees would have developed resistance to the similar level.
The other finding was that the Korean population of Apis mellifera showed much higher LC50 values for the selected insecticides. The LC50 of carbaryl which is the carbamate insecticide which had been used in agricultural field more than 50 years, showed only a 5 times difference. But the newer neonicotinoid insecticides were about 100 times different. The pattern was similar to the use of pesticide and fertilizer in both countries. However, pesticide and fertilizer use (kg/ha) was 3-6 times higher in Korea than in Thailand. The relationship between the pesticide use and tolerance of non-target beneficial organisms would be required. There are some reports of the different toxicities of insecticides to different honeybees. Colony development and worker honeybee physiological conditions change during the season (Winston, 1987). Even when using A. mellifera, substantial differences often emerge when results of toxicity tests on either different genotypes of honeybees or the same honeybee species but different laboratories are compared (Laurino et al., 2013). Large differences in the temperature at which the tests are carried out, the age of the honeybees used in the tests, and in the way honeybees are processed and dosed with the toxic substances can lead to substantially different results between different laboratories, even if the same guide lines are followed (Ladas, 1972; Aupinel et al., 2009; Medrzycki et al., 2012). However in our experiments, every step was standardized and performed by the same persons which partially reduced the chance of errors.
Even though neonicotinoid insecticides are considered less harmful to animals and humans, a range of studies have been done to assess adverse ecological effects, including honey-bee colony collapse disorder (CCD) and losses of birds. In 2013, the European Union and a few non-EU countries restricted the use of certain neonicotinioids (Cressey 2013). Even with the increasing concerns on the toxicity of neonicotinoid pesticides, they are still the most prevalent group of pesticides used to control various agricultural arthropod pests (Cresswell, 2011). Kang and Jung (2010) reported that most honeybee damage is associated with insecticides rather than fungicides or acaricides. Also, even with the same pesticide, toxicities were different to bumblebees and honeybees. A carbaryl residual study on B. terrestris and A. mellifera showed no toxic effect on bumblebee but highly toxic effects to honeybees (Kim and Jung, 2013a). A recent questionnaire study on honeybee poisoning incidents from beekeepers and apple growers in Korea revealed interesting arguments (Kang and Jung, 2010; Kim and Jung, 2013b). In Korea, RDA (Rural Develop-ment Administration) is the authority for the registration, regulation and safety management of plant protecting products including pesticides, following the pesticide management Act. Careful monitoring of the pesticide use in conjunction with the field risk assessments are required. Also much of the indepth information on the proper use of pesticides during floral blooming was propagated though the agricultural extension and pesticide company marketing teams. Also, reevaluating the hazards they imp-osed (e.g., toxicity information such as LC50, LD50, or hazard quotients (HQ)) could be the primary step, but should expand to the risk assessment in more realistic conditions, which means the environmental monitoring of the pesticides used in agricultural production with emphases on those concerned chemicals.
Acknowledgments
This study was partly supported from the RDA agenda of PJ00997004. We also thank Dr. Yaowaluk Chanbang, of the Chiang Mai University, Department of Entomology and Plant Pathology, for arranging laboratory space during the pesticide evaluation phase of this work at CMU.
References
-
Aizen, M.A., and L.D. Harder, (2009), The global stock of domesticated honey bees is growing slower than agricultural demand for pollination, Current Biology, 19, p915-918.
[https://doi.org/10.1016/j.cub.2009.03.071]
- Atkins, P.W., (1992), General chemistry, Scientific American Books, New York.
- Aupinel, P., D. Fortini, B. Michaud, P. Medrzycki, E. Padovani, D. Przygoda, C. Maus, J.D. Charriere, V. Kilchenmann, R. Galle, J.J. Vollmann, L. Jeker, M. Janke, J.F. Odoux, and J. Tasei, (2009), Honey bee brood ring-test: method for testing pesticide toxicity on honey bee brood in laboratory conditions, Julius-Kuhn- Archiv, 423.
-
Colla, S.R., M.C. Otterstatter, R.J. Gegear, and J.D. Thomson, (2006), Plight of the bumble bee: pathogen spillover from commercial to wild population, Biological Conservation, 129, p461-467.
[https://doi.org/10.1016/j.biocon.2005.11.013]
-
Cordes, N., W. Huang, J.P. Strange, S.A. Cameron, T.L. Griswold, J.D. Lozier, and L.F. Solter, (2012), Interspecific geographic distribution and variation of the pathogens Nosema bombi and Crithidia species in United States bumble bee populations, Journal of Invertebrate Pathology, 109, p209-216.
[https://doi.org/10.1016/j.jip.2011.11.005]
-
Cresswell, J.E., (2011), A meta-analysis of experiments testing the effectsof a neonicotinoid insecticide (imidacloprid) on honey bees, Ecotoxicology, 20, p149-157.
[https://doi.org/10.1007/s10646-010-0566-0]
-
Cressey, D., (2013), Europe debates risk to bees, Nature, 496(7446), p408.
[https://doi.org/10.1038/496408a]
-
Frust, M.A., D.P. McMahon, J.L. Osborne, R.J. Paxton, and M.J.F. Brown, (2014), Disease associations between honeybees and bumblebees as a threat to wild pollinators, Nature, 506, p364-366.
[https://doi.org/10.1038/nature12977]
-
Goulson, D., (2003), Effects of introduced bees on native ecosystems, Annual Review of Ecology, Evolution and Systematics, 34, p1-26.
[https://doi.org/10.1146/annurev.ecolsys.34.011802.132355]
-
Graystock, P., K. Yates, S.E.F. Evison, B. Darvill, D. Goulson, and W.O.H. Hughes, (2015), The Trojan hives: pollinator pathogens, imported and distributed in bumblebee colonies, Journal of Applied Ecology, 50, p1207-1215.
[https://doi.org/10.1111/1365-2664.12134]
- Jung, C., (2008), Economic value of honeybee pollination on major frit and vegetable crops in Korea, Korean J. Apic, 23, p147-152.
-
Jung, C., and S. Cho, (2015), Relationship between honeybee population and honey production in Korea: A historical trend analysis, Korean J. Apic, 30, p7-12.
[https://doi.org/10.17519/apiculture.2015.04.30.1.7]
-
Kevan, P.G., (1999), Pollinators as bio-indicators of the state of environment: species, activity and diversity, Agriculture Ecosystem and Environment, 74, p373-393.
[https://doi.org/10.1016/S0167-8809(99)00044-4]
- Kim, D., and C. Jung, (2013b), Comparative analysis the perception on honeybee damage by the pesticide between beekeepers and apple growers, Korean J. Apic, 28, p33-38.
- Kim, D., and C. Jung, (2013a), Residual Toxicity of Carbaryl and Lime Sulfur on the European Honey Bee, Apis mellifera (Hymenoptera: Apidae) and Buff-tailed Bumble Bee, Bombus terrestris (Hymenoptera: Apidae), Korean J. Apic, 28, p25-32.
-
Klein, A-M., B.E. Vaissiere, J.H. Cane, I. Steffan-Dewenter, S.A. Cunnigham, C. Kremen, and TT. Tscharntke, (2007), Importance of pollinators in changing landscapes for world crops, Proc. R. Soc. B, 274, p303-313.
[https://doi.org/10.1098/rspb.2006.3721]
- Kluser, S., and P. Peduzzi, (2007), Global pollinator decline: A literature review, United Nations Environment Programme.
-
Ladas, A., (1972), Der einfluss verschiedener konstitutions- und umweltfaktoren auf die anfã’’lligkeit der honigbiene (Apis mellifica l.) gegenãceber zwei insektiziden pflanzenschutzmitteln, Apidologie, 3, p55-78.
[https://doi.org/10.1051/apido:19720103]
- Laurino, D., (2013), Toxicity of neonicotinoid insecticides on different honey bee genotypes, Bulletin of Insectology, 66, p119-126.
-
Lebuhn, G., D. Sam, E.F. Connor, B. Gemill-Herren, S.G. Potts, R.L. Mincley, T. Griswold, R. Jean, E. K, D.W. Roubik, J. Cane, K.W. Wright, G. Frankie, and F. Parker, (2012), Detecting insect pollinator declines on regional and global scales, Conservation Biology, 27, p113-120.
[https://doi.org/10.1111/j.1523-1739.2012.01962.x]
- McGregor, S.E., (1976), Insect pollination of cultivated crop plants. U.S. Department of Agriculture-Agricultural Research Service, Washington, DC.
- Medrzycki, P., F. Sgolastra, G. bogo, S. Tosi, and S. Venturi, (2012), Influence of some experimental conditions on the results of laboratory toxicological test on honeybees, Julius Kuhn-Archiv, 437, p104.
- Oldroyd, B.P., and S. Wongsiri, (2006), Asian honey bees. Biology, conservation and human interactions, Harvard University Press, London, England, p339.
-
Rand, E. E. du., S. Smit, M. Beukes, Z. Apostolides, C.W.W. Pirk, and S.W. Nicolson, (2015), Detoxification mechanisms of honey bees (Apis mellifera) resulting in tolerance of dietary nicotine, Scientific Reports, 5, p11779.
[https://doi.org/10.1038/srep11779]
-
Roubik, D.W., and S.L. Buchmann, (1984), Nectar selection by Melipona and Apis mellifera (Hymenoptera: Apidae) and the ecology of nectar intake by bee colonies in a tropical forest, Oecologia (Berlin), 61, p1-10.
[https://doi.org/10.1007/BF00379082]
-
Scheiner, R., R.E. Page, and J. Erber, (2004), Sucrose responsiveness and behavioral plasticity in honey bees (Apis mellifera), Apidologie, 35, p133-142.
[https://doi.org/10.1051/apido:2004001]
- Suh, W.T., and J.H. Shim, (1989), A study on the enzyme activities of a honeybee (Apis cerana F.) associated with the degradation of some insecticides. Korean, J. Environ. Agric, 8, p47-54.
-
Thomas, C.D., A. Cameron, R.E. Green, M. Bakkenes, L.J. Beaumont, Y.C. Collingham, B.F.N. Erasmus, M.F. de. Siqueira, A. Grainger, L. Hannah, L. Hughes, B. Huntley, A.S. van. Jaarsveld, G.F. Midgley, L. Miles, M.A. Ortega-Huerta, A.T. Peterson, O.L. Phillips, and S.E. Williams, (2004), Extinction risk from climate change, Nature, 427, p145-148.
[https://doi.org/10.1038/nature02121]
- Winston, M.L., (1987), The Biology of the Honey Bee, Harvard Univ. Press, Cambridge, MA.
-
Yamamoto, I., (1999), Nicotine to neonicotinoids: 1962 to 1997, in Nicotinoid Insecticides and the Nicotinic Acetylcholine Receptor, Yamamoto, I., and Casida, J.E. eds, p3-27, Springer-Verlag, Tokyo.
[https://doi.org/10.1007/978-4-431-67933-2_1]